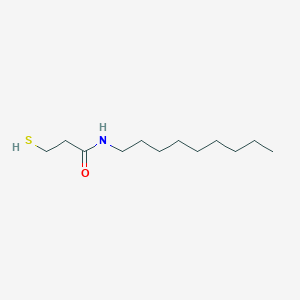
3-Mercapto-N-nonylpropionamide
Description
3-Mercapto-N-nonylpropionamide is a useful research compound. Its molecular formula is C12H25NOS and its molecular weight is 231.4 g/mol. The purity is usually 95%.
BenchChem offers high-quality this compound suitable for many research applications. Different packaging options are available to accommodate customers' requirements. Please inquire for more information about this compound including the price, delivery time, and more detailed information at info@benchchem.com.
Properties
IUPAC Name |
N-nonyl-3-sulfanylpropanamide | |
---|---|---|
Source | PubChem | |
URL | https://pubchem.ncbi.nlm.nih.gov | |
Description | Data deposited in or computed by PubChem | |
InChI |
InChI=1S/C12H25NOS/c1-2-3-4-5-6-7-8-10-13-12(14)9-11-15/h15H,2-11H2,1H3,(H,13,14) | |
Source | PubChem | |
URL | https://pubchem.ncbi.nlm.nih.gov | |
Description | Data deposited in or computed by PubChem | |
InChI Key |
CKRXZOZYQYDWIQ-UHFFFAOYSA-N | |
Source | PubChem | |
URL | https://pubchem.ncbi.nlm.nih.gov | |
Description | Data deposited in or computed by PubChem | |
Canonical SMILES |
CCCCCCCCCNC(=O)CCS | |
Source | PubChem | |
URL | https://pubchem.ncbi.nlm.nih.gov | |
Description | Data deposited in or computed by PubChem | |
Molecular Formula |
C12H25NOS | |
Source | PubChem | |
URL | https://pubchem.ncbi.nlm.nih.gov | |
Description | Data deposited in or computed by PubChem | |
DSSTOX Substance ID |
DTXSID10693508 | |
Record name | N-Nonyl-3-sulfanylpropanamide | |
Source | EPA DSSTox | |
URL | https://comptox.epa.gov/dashboard/DTXSID10693508 | |
Description | DSSTox provides a high quality public chemistry resource for supporting improved predictive toxicology. | |
Molecular Weight |
231.40 g/mol | |
Source | PubChem | |
URL | https://pubchem.ncbi.nlm.nih.gov | |
Description | Data deposited in or computed by PubChem | |
CAS No. |
228716-16-3 | |
Record name | N-Nonyl-3-sulfanylpropanamide | |
Source | EPA DSSTox | |
URL | https://comptox.epa.gov/dashboard/DTXSID10693508 | |
Description | DSSTox provides a high quality public chemistry resource for supporting improved predictive toxicology. | |
Retrosynthesis Analysis
AI-Powered Synthesis Planning: Our tool employs the Template_relevance Pistachio, Template_relevance Bkms_metabolic, Template_relevance Pistachio_ringbreaker, Template_relevance Reaxys, Template_relevance Reaxys_biocatalysis model, leveraging a vast database of chemical reactions to predict feasible synthetic routes.
One-Step Synthesis Focus: Specifically designed for one-step synthesis, it provides concise and direct routes for your target compounds, streamlining the synthesis process.
Accurate Predictions: Utilizing the extensive PISTACHIO, BKMS_METABOLIC, PISTACHIO_RINGBREAKER, REAXYS, REAXYS_BIOCATALYSIS database, our tool offers high-accuracy predictions, reflecting the latest in chemical research and data.
Strategy Settings
Precursor scoring | Relevance Heuristic |
---|---|
Min. plausibility | 0.01 |
Model | Template_relevance |
Template Set | Pistachio/Bkms_metabolic/Pistachio_ringbreaker/Reaxys/Reaxys_biocatalysis |
Top-N result to add to graph | 6 |
Feasible Synthetic Routes
Q1: How does 3-Mercapto-N-nonylpropionamide interact with gold surfaces and what are the downstream effects of this interaction?
A1: this compound forms self-assembled monolayers (SAMs) on gold surfaces, primarily through the strong interaction between the sulfur atom of the thiol group and the gold atoms. [, ] This interaction leads to the formation of a well-ordered monolayer with the alkyl chains oriented away from the surface. The presence of the amide group within the alkyl chain introduces an additional level of organization due to the formation of hydrogen bonds between neighboring molecules. [, , ] These hydrogen bonds contribute to the stability of the SAM and influence its structure.
Q2: How do hydrogen bonds influence the structure of this compound SAMs?
A2: Hydrogen bonding between the amide groups of adjacent molecules plays a crucial role in the structural organization of this compound SAMs. [, ] These interactions lead to the formation of linear hydrogen-bond networks that extend across the monolayer, influencing both the packing density and the orientation of the molecules. [] Studies have shown that these networks can even cross the boundaries between domains with different rotational orientations. []
Q3: Can the structure of this compound SAMs be manipulated?
A3: Yes, the structure of this compound SAMs can be manipulated by electrochemical processing. [] Applying a specific electrochemical potential can induce a phase transition within the monolayer, transforming a close-packed structure into a striped phase. [] This transition is attributed to the disruption of the hydrogen-bonding network between amide groups during the electrochemical desorption process. []
Q4: Does this compound exhibit phase separation when co-assembled with other alkanethiols?
A4: Yes, this compound displays phase separation when co-assembled with other alkanethiols, even those with similar chain lengths. [] This behavior contrasts with mixtures of n-alkanethiols, which typically do not exhibit phase separation. [] The presence of the hydrogen-bonding amide group within this compound is believed to drive this phase separation, leading to the formation of single-component domains on the nanometer scale. []
Disclaimer and Information on In-Vitro Research Products
Please be aware that all articles and product information presented on BenchChem are intended solely for informational purposes. The products available for purchase on BenchChem are specifically designed for in-vitro studies, which are conducted outside of living organisms. In-vitro studies, derived from the Latin term "in glass," involve experiments performed in controlled laboratory settings using cells or tissues. It is important to note that these products are not categorized as medicines or drugs, and they have not received approval from the FDA for the prevention, treatment, or cure of any medical condition, ailment, or disease. We must emphasize that any form of bodily introduction of these products into humans or animals is strictly prohibited by law. It is essential to adhere to these guidelines to ensure compliance with legal and ethical standards in research and experimentation.