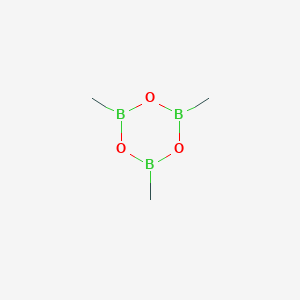
Trimethylboroxine
Overview
Description
It is a colorless to yellow solution with the molecular formula C₃H₉B₃O₃ and a molecular weight of 125.53 g/mol . This compound is known for its versatility in various chemical reactions and applications, making it a valuable reagent in scientific research and industrial processes.
Mechanism of Action
Target of Action
Trimethylboroxine (TMB) is a cyclic anhydride of methyl-boronic acid . Its primary targets are various aromatic halides and C(sp3)−H bonds . These targets play a crucial role in the methylation process, which is fundamental in medicinal and material chemistry .
Mode of Action
TMB interacts with its targets through a palladium-catalyzed Suzuki-Miyaura coupling reaction . This reaction involves the transfer of a methyl group from TMB to the target molecule, resulting in the methylation of the target .
Biochemical Pathways
The biochemical pathway primarily affected by TMB is the methylation pathway . Methylation is a key process in biological molecules, pharmaceuticals, and organic functional materials . The downstream effects of this pathway include alterations in the physical properties and biological activity of molecules, including solubility, hydrophilicity, half-life, and conformation of drugs .
Pharmacokinetics
Its impact on bioavailability is significant due to its role as a methylating agent .
Result of Action
The molecular and cellular effects of TMB’s action primarily involve the methylation of various aromatic halides and C(sp3)−H bonds . This methylation can dramatically alter both the physical properties and biological activity of molecules .
Biochemical Analysis
Biochemical Properties
Trimethylboroxine serves as a robust electron acceptor and exhibits oxidizing properties in biochemical reactions . It can also function as a catalyst in select chemical reactions . In biochemical processes, this compound acts as an electron acceptor, facilitating electron transfer from one molecule to another . This unique capability empowers this compound to catalyze biochemical reactions effectively .
Molecular Mechanism
It is known to act as a methylating reagent in the methylation of various aromatic halides . This suggests that this compound may interact with biomolecules through methylation, potentially influencing gene expression and enzyme activity.
Temporal Effects in Laboratory Settings
It is known that this compound is stable, allowing for long-term storage .
Preparation Methods
Trimethylboroxine is typically prepared by heating trimethylborane (B(CH₃)₃) and boron trioxide (B₂O₃) together in a sealed tube . The boron trioxide powder is made by dehydrating boric acid (H₃BO₃) under vacuum over phosphorus pentoxide (P₂O₅) at 220°C . This method ensures the exclusion of moisture, which is crucial as this compound is highly hygroscopic.
Chemical Reactions Analysis
Trimethylboroxine undergoes various chemical reactions, including:
Methylation: It acts as a methylating agent for the methylation of aromatic halides and C(sp³)-H bonds using palladium catalysts.
Derivatization: It is used as a derivatizing agent for gas chromatographic/mass spectrometric analysis.
Polymerization: It is involved in the preparation of methylaluminoxane (MAO), which is used in the polymerization of olefins.
Electrolyte Additive: It enhances the interface stability of electrode/electrolyte systems.
Common reagents and conditions used in these reactions include palladium catalysts, dichloromethane, and tetrahydrofuran (THF) . Major products formed from these reactions include methylated aromatic compounds and polymer-supported catalysts .
Scientific Research Applications
Trimethylboroxine has a wide range of applications in scientific research:
Comparison with Similar Compounds
Compared to these compounds, trimethylboroxine is unique due to its high reactivity and versatility in methylation reactions . Other similar compounds include:
Triphenylboroxine: Used in the synthesis of boron-containing polymers.
Tris(trimethylsilyl)borate: Employed as a reagent in organic synthesis.
Trimethyl borate: Utilized in the production of boron-containing compounds.
This compound stands out for its ability to efficiently methylate a wide range of substrates, making it a valuable tool in both academic and industrial research .
Biological Activity
Trimethylboroxine (TMB), with the chemical formula , is a cyclic anhydride derivative of methyl-boronic acid. It has garnered attention in both organic chemistry and biological applications due to its unique properties as a methylating agent. This article delves into the biological activity of TMB, highlighting its mechanisms, applications, and relevant research findings.
Chemical Structure and Stability
this compound is typically presented as a colorless to yellow liquid, with a molecular weight of 125.53 g/mol. It is completely soluble in non-nucleophilic solvents, which enhances its utility in various chemical reactions . TMB is stable under laboratory conditions, allowing for long-term storage without significant degradation.
Mechanism of Action
TMB acts primarily as a methylating agent through palladium-catalyzed Suzuki-Miyaura coupling reactions. This mechanism allows TMB to methylate challenging substrates, including aryl electrophiles that are typically resistant to conventional methylation methods . The compound's ability to facilitate the methylation of various aromatic halides and C(sp³)-H bonds significantly impacts its biological activity by modifying the physical properties and reactivity of the target molecules .
Biological Applications
TMB's role as a methylating agent extends into various fields, including medicinal chemistry, where it has potential applications in drug development and synthesis.
Pharmaceutical Chemistry
In pharmaceutical applications, TMB has been utilized to methylate complex aryl compounds that are often difficult to modify using traditional methods. For instance, researchers have successfully employed TMB to methylate nitroarenes and benzoic acids using palladium catalysts, expanding the scope of available pharmaceutical intermediates . This versatility makes TMB a valuable reagent in the synthesis of novel drug candidates.
Biochemical Pathways
The primary biochemical pathway affected by TMB is the methylation pathway. Methylation can alter gene expression and protein function, thereby influencing various biological processes. The incorporation of methyl groups into biomolecules can enhance their stability and bioactivity, which is crucial for developing effective therapeutic agents .
Table 1: Comparison of Methylation Reagents
Reagent | Reactivity | Applications |
---|---|---|
This compound | High | Methylation of challenging substrates |
Trimethyl borate | Moderate | General methylation |
Triphenylboroxine | Low | Synthesis of boron-containing polymers |
Case Study: Methylation of Nitroarenes
A study conducted by Feng et al. demonstrated the effectiveness of TMB in methylating nitroarenes using Pd(acac)₂ as a catalyst. The reaction conditions allowed for high yields of the corresponding methylated products, showcasing TMB's ability to overcome traditional limitations in aryl electrophile methylation .
Table 2: Yields from Methylation Reactions
Substrate Type | Yield (%) | Catalyst Used |
---|---|---|
Nitroarenes | 85-95 | Pd(acac)₂ |
Benzoic Acids | 80-90 | Pd(OAc)₂ |
Properties
IUPAC Name |
2,4,6-trimethyl-1,3,5,2,4,6-trioxatriborinane | |
---|---|---|
Source | PubChem | |
URL | https://pubchem.ncbi.nlm.nih.gov | |
Description | Data deposited in or computed by PubChem | |
InChI |
InChI=1S/C3H9B3O3/c1-4-7-5(2)9-6(3)8-4/h1-3H3 | |
Source | PubChem | |
URL | https://pubchem.ncbi.nlm.nih.gov | |
Description | Data deposited in or computed by PubChem | |
InChI Key |
GBBSAMQTQCPOBF-UHFFFAOYSA-N | |
Source | PubChem | |
URL | https://pubchem.ncbi.nlm.nih.gov | |
Description | Data deposited in or computed by PubChem | |
Canonical SMILES |
B1(OB(OB(O1)C)C)C | |
Source | PubChem | |
URL | https://pubchem.ncbi.nlm.nih.gov | |
Description | Data deposited in or computed by PubChem | |
Molecular Formula |
C3H9B3O3 | |
Source | PubChem | |
URL | https://pubchem.ncbi.nlm.nih.gov | |
Description | Data deposited in or computed by PubChem | |
DSSTOX Substance ID |
DTXSID90341570 | |
Record name | Trimethylboroxine | |
Source | EPA DSSTox | |
URL | https://comptox.epa.gov/dashboard/DTXSID90341570 | |
Description | DSSTox provides a high quality public chemistry resource for supporting improved predictive toxicology. | |
Molecular Weight |
125.5 g/mol | |
Source | PubChem | |
URL | https://pubchem.ncbi.nlm.nih.gov | |
Description | Data deposited in or computed by PubChem | |
CAS No. |
823-96-1 | |
Record name | Trimethylboroxin | |
Source | CAS Common Chemistry | |
URL | https://commonchemistry.cas.org/detail?cas_rn=823-96-1 | |
Description | CAS Common Chemistry is an open community resource for accessing chemical information. Nearly 500,000 chemical substances from CAS REGISTRY cover areas of community interest, including common and frequently regulated chemicals, and those relevant to high school and undergraduate chemistry classes. This chemical information, curated by our expert scientists, is provided in alignment with our mission as a division of the American Chemical Society. | |
Explanation | The data from CAS Common Chemistry is provided under a CC-BY-NC 4.0 license, unless otherwise stated. | |
Record name | Trimethylboroxine | |
Source | EPA DSSTox | |
URL | https://comptox.epa.gov/dashboard/DTXSID90341570 | |
Description | DSSTox provides a high quality public chemistry resource for supporting improved predictive toxicology. | |
Retrosynthesis Analysis
AI-Powered Synthesis Planning: Our tool employs the Template_relevance Pistachio, Template_relevance Bkms_metabolic, Template_relevance Pistachio_ringbreaker, Template_relevance Reaxys, Template_relevance Reaxys_biocatalysis model, leveraging a vast database of chemical reactions to predict feasible synthetic routes.
One-Step Synthesis Focus: Specifically designed for one-step synthesis, it provides concise and direct routes for your target compounds, streamlining the synthesis process.
Accurate Predictions: Utilizing the extensive PISTACHIO, BKMS_METABOLIC, PISTACHIO_RINGBREAKER, REAXYS, REAXYS_BIOCATALYSIS database, our tool offers high-accuracy predictions, reflecting the latest in chemical research and data.
Strategy Settings
Precursor scoring | Relevance Heuristic |
---|---|
Min. plausibility | 0.01 |
Model | Template_relevance |
Template Set | Pistachio/Bkms_metabolic/Pistachio_ringbreaker/Reaxys/Reaxys_biocatalysis |
Top-N result to add to graph | 6 |
Feasible Synthetic Routes
Disclaimer and Information on In-Vitro Research Products
Please be aware that all articles and product information presented on BenchChem are intended solely for informational purposes. The products available for purchase on BenchChem are specifically designed for in-vitro studies, which are conducted outside of living organisms. In-vitro studies, derived from the Latin term "in glass," involve experiments performed in controlled laboratory settings using cells or tissues. It is important to note that these products are not categorized as medicines or drugs, and they have not received approval from the FDA for the prevention, treatment, or cure of any medical condition, ailment, or disease. We must emphasize that any form of bodily introduction of these products into humans or animals is strictly prohibited by law. It is essential to adhere to these guidelines to ensure compliance with legal and ethical standards in research and experimentation.