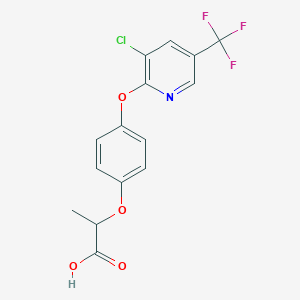
Haloxyfop
Overview
Description
Haloxyfop is a selective herbicide belonging to the aryloxyphenoxypropionic acid family. It is primarily used to control annual and perennial grass weeds in various broad-leaf crops such as sugar beet, oilseed, potatoes, leaf vegetables, onions, sunflowers, and strawberries . This compound is available in different forms, including this compound-methyl and this compound-ethoxyethyl .
Mechanism of Action
Target of Action
Haloxyfop, a selective aryloxyphenoxypropionate (AOPP) herbicide, primarily targets the enzyme Acetyl-CoA carboxylase 2 (ACCase 2) in plants . ACCase is a crucial enzyme involved in lipid metabolism, catalyzing the ATP-dependent carboxylation of acetyl-CoA to malonyl-CoA .
Mode of Action
This compound interacts with its target, ACCase, by inhibiting its activity . This inhibition disrupts the synthesis of fatty acids, which are essential components of phospholipids required for cell membrane development and cell growth . The inhibition of ACCase leads to the cessation of growth, discoloration, and eventual necrosis of plant tissue .
Biochemical Pathways
The primary biochemical pathway affected by this compound is the lipid metabolism pathway . By inhibiting ACCase, this compound disrupts the conversion of acetyl-CoA to malonyl-CoA, a critical step in the biosynthesis of fatty acids . This disruption affects the production of phospholipids necessary for cell membrane development, leading to growth cessation and eventual plant death .
Pharmacokinetics
Upon application to a plant, the esters of this compound are quickly broken down to release free acid, which is readily translocated throughout the plant . The compound becomes conjugated, typically as glycosides, which are polar . It’s known that this compound has a low volatility and is slightly soluble in water .
Result of Action
The molecular effect of this compound involves the inhibition of the ACCase enzyme, leading to a disruption in fatty acid synthesis . On a cellular level, this results in the cessation of growth, discoloration, and eventual necrosis of plant tissue . In some cases, resistance to this compound has been observed, associated with a target site mutation in the ACCase enzyme .
Action Environment
Environmental factors can influence the action of this compound. For instance, water stress conditions can reduce the herbicide’s injury to plants, attributed to a reduction in herbicide translocation and spray retention, or changes in the metabolism of the herbicide . Furthermore, the persistence of this compound in the soil and its degradation by bacteria contribute to its environmental degradation .
Biochemical Analysis
Biochemical Properties
Haloxyfop plays a significant role in biochemical reactions by inhibiting the enzyme acetyl-CoA carboxylase (ACCase). This enzyme is essential for the carboxylation of acetyl-CoA to malonyl-CoA, a critical step in fatty acid biosynthesis. By inhibiting ACCase, this compound disrupts the production of fatty acids, leading to the cessation of cell membrane synthesis and ultimately causing cell death in susceptible plants . This compound interacts with the ACCase enzyme by binding to its carboxyltransferase domain, preventing the enzyme from catalyzing its reaction .
Cellular Effects
This compound exerts profound effects on various types of cells and cellular processes. In plant cells, it inhibits cell division and elongation by disrupting fatty acid synthesis, which is vital for cell membrane formation . This inhibition leads to the accumulation of metabolic intermediates and a decline in cellular ATP levels . In animal cells, this compound has been shown to affect cell signaling pathways, gene expression, and cellular metabolism by interfering with lipid biosynthesis . The compound’s impact on cell function includes alterations in the uptake and utilization of metabolic intermediates, leading to reduced cell viability and growth .
Molecular Mechanism
The molecular mechanism of this compound involves its binding to the carboxyltransferase domain of the acetyl-CoA carboxylase enzyme, inhibiting its activity . This binding prevents the conversion of acetyl-CoA to malonyl-CoA, a crucial step in fatty acid biosynthesis . The inhibition of ACCase by this compound leads to a reduction in fatty acid production, disrupting cell membrane synthesis and causing cell death in susceptible plants . Additionally, this compound has been shown to induce changes in gene expression related to lipid metabolism and stress responses .
Temporal Effects in Laboratory Settings
In laboratory settings, the effects of this compound change over time due to its stability and degradation. This compound is relatively stable under neutral and acidic conditions but degrades rapidly under alkaline conditions . Over time, the compound’s efficacy may decrease due to its degradation, leading to reduced herbicidal activity . Long-term studies have shown that this compound can cause persistent changes in cellular function, including alterations in lipid metabolism and cell viability . These effects are observed in both in vitro and in vivo studies, highlighting the compound’s potential for long-term impact on cellular processes .
Dosage Effects in Animal Models
The effects of this compound vary with different dosages in animal models. At low doses, this compound has minimal impact on cellular function and overall health . At higher doses, the compound can cause significant toxic effects, including liver and kidney damage, reduced growth rates, and alterations in lipid metabolism . Threshold effects have been observed, where a specific dosage level leads to a marked increase in adverse effects . These findings underscore the importance of careful dosage management when using this compound in agricultural settings .
Metabolic Pathways
This compound is involved in several metabolic pathways, primarily related to its role as an inhibitor of acetyl-CoA carboxylase . The compound is metabolized in plants and animals through hydrolysis, leading to the formation of various metabolites . These metabolites can further interact with enzymes and cofactors involved in lipid metabolism, affecting metabolic flux and metabolite levels . The primary metabolic pathway of this compound involves its conversion to this compound acid, which retains herbicidal activity .
Transport and Distribution
Within cells and tissues, this compound is transported and distributed through various mechanisms. The compound is absorbed by plant roots and leaves, where it is translocated to other parts of the plant . In animal models, this compound is distributed to various tissues, including the liver, kidneys, and fat . Transporters and binding proteins play a crucial role in the localization and accumulation of this compound within cells . The compound’s distribution is influenced by its chemical properties, including solubility and stability .
Subcellular Localization
This compound’s subcellular localization is primarily within the chloroplasts of plant cells, where it exerts its herbicidal activity by inhibiting acetyl-CoA carboxylase . The compound’s localization is directed by targeting signals and post-translational modifications that ensure its delivery to specific compartments within the cell . In animal cells, this compound is localized to various organelles involved in lipid metabolism, including the endoplasmic reticulum and mitochondria . This subcellular localization is critical for the compound’s activity and function, as it allows this compound to interact with its target enzymes and exert its effects on cellular processes .
Preparation Methods
Haloxyfop can be synthesized through several methods. One common approach involves the esterification of this compound acid with methanol or ethoxyethanol to produce this compound-methyl or this compound-ethoxyethyl, respectively . The preparation process typically includes steps such as alkaline hydrolysis, extraction with acetonitrile, and phase separation using magnesium sulfate and sodium chloride . Industrial production methods often involve the use of catalysts like tetrabutylammonium chloride and controlled temperature conditions .
Chemical Reactions Analysis
Haloxyfop undergoes various chemical reactions, including hydrolysis and oxidation. For instance, this compound-methyl can be hydrolyzed to release this compound acid . Common reagents used in these reactions include methanolic sodium hydroxide for hydrolysis and acetonitrile for extraction . The major products formed from these reactions are the parent acid and its esters .
Scientific Research Applications
Haloxyfop has several scientific research applications. It is widely used in agricultural research to study weed resistance and herbicide efficacy . In analytical chemistry, this compound is used to develop and validate methods for detecting pesticide residues in various matrices, including infant formulas . Additionally, this compound’s ability to inhibit lipid synthesis makes it a valuable tool in studying metabolic pathways and enzyme functions in plants .
Comparison with Similar Compounds
Haloxyfop is similar to other aryloxyphenoxypropionic acid herbicides, such as fluazifop and quizalofop. These compounds share a similar mode of action, targeting the acetyl-CoA carboxylase enzyme . this compound is unique in its high efficacy against a broad range of grass weeds and its ability to be used in various crop types . The similar compounds include:
- Fluazifop
- Quizalofop
- Diclofop
Properties
IUPAC Name |
2-[4-[3-chloro-5-(trifluoromethyl)pyridin-2-yl]oxyphenoxy]propanoic acid | |
---|---|---|
Source | PubChem | |
URL | https://pubchem.ncbi.nlm.nih.gov | |
Description | Data deposited in or computed by PubChem | |
InChI |
InChI=1S/C15H11ClF3NO4/c1-8(14(21)22)23-10-2-4-11(5-3-10)24-13-12(16)6-9(7-20-13)15(17,18)19/h2-8H,1H3,(H,21,22) | |
Source | PubChem | |
URL | https://pubchem.ncbi.nlm.nih.gov | |
Description | Data deposited in or computed by PubChem | |
InChI Key |
GOCUAJYOYBLQRH-UHFFFAOYSA-N | |
Source | PubChem | |
URL | https://pubchem.ncbi.nlm.nih.gov | |
Description | Data deposited in or computed by PubChem | |
Canonical SMILES |
CC(C(=O)O)OC1=CC=C(C=C1)OC2=C(C=C(C=N2)C(F)(F)F)Cl | |
Source | PubChem | |
URL | https://pubchem.ncbi.nlm.nih.gov | |
Description | Data deposited in or computed by PubChem | |
Molecular Formula |
C15H11ClF3NO4 | |
Source | PubChem | |
URL | https://pubchem.ncbi.nlm.nih.gov | |
Description | Data deposited in or computed by PubChem | |
Related CAS |
69806-86-6 (hydrochloride salt) | |
Record name | Haloxyfop [ANSI:BSI:ISO] | |
Source | ChemIDplus | |
URL | https://pubchem.ncbi.nlm.nih.gov/substance/?source=chemidplus&sourceid=0069806344 | |
Description | ChemIDplus is a free, web search system that provides access to the structure and nomenclature authority files used for the identification of chemical substances cited in National Library of Medicine (NLM) databases, including the TOXNET system. | |
DSSTOX Substance ID |
DTXSID7042019 | |
Record name | Haloxyfop | |
Source | EPA DSSTox | |
URL | https://comptox.epa.gov/dashboard/DTXSID7042019 | |
Description | DSSTox provides a high quality public chemistry resource for supporting improved predictive toxicology. | |
Molecular Weight |
361.70 g/mol | |
Source | PubChem | |
URL | https://pubchem.ncbi.nlm.nih.gov | |
Description | Data deposited in or computed by PubChem | |
CAS No. |
69806-34-4 | |
Record name | Haloxyfop | |
Source | CAS Common Chemistry | |
URL | https://commonchemistry.cas.org/detail?cas_rn=69806-34-4 | |
Description | CAS Common Chemistry is an open community resource for accessing chemical information. Nearly 500,000 chemical substances from CAS REGISTRY cover areas of community interest, including common and frequently regulated chemicals, and those relevant to high school and undergraduate chemistry classes. This chemical information, curated by our expert scientists, is provided in alignment with our mission as a division of the American Chemical Society. | |
Explanation | The data from CAS Common Chemistry is provided under a CC-BY-NC 4.0 license, unless otherwise stated. | |
Record name | Haloxyfop [ANSI:BSI:ISO] | |
Source | ChemIDplus | |
URL | https://pubchem.ncbi.nlm.nih.gov/substance/?source=chemidplus&sourceid=0069806344 | |
Description | ChemIDplus is a free, web search system that provides access to the structure and nomenclature authority files used for the identification of chemical substances cited in National Library of Medicine (NLM) databases, including the TOXNET system. | |
Record name | Haloxyfop | |
Source | EPA DSSTox | |
URL | https://comptox.epa.gov/dashboard/DTXSID7042019 | |
Description | DSSTox provides a high quality public chemistry resource for supporting improved predictive toxicology. | |
Record name | HALOXYFOP | |
Source | FDA Global Substance Registration System (GSRS) | |
URL | https://gsrs.ncats.nih.gov/ginas/app/beta/substances/24TYO6H90H | |
Description | The FDA Global Substance Registration System (GSRS) enables the efficient and accurate exchange of information on what substances are in regulated products. Instead of relying on names, which vary across regulatory domains, countries, and regions, the GSRS knowledge base makes it possible for substances to be defined by standardized, scientific descriptions. | |
Explanation | Unless otherwise noted, the contents of the FDA website (www.fda.gov), both text and graphics, are not copyrighted. They are in the public domain and may be republished, reprinted and otherwise used freely by anyone without the need to obtain permission from FDA. Credit to the U.S. Food and Drug Administration as the source is appreciated but not required. | |
Synthesis routes and methods I
Procedure details
Synthesis routes and methods II
Procedure details
Retrosynthesis Analysis
AI-Powered Synthesis Planning: Our tool employs the Template_relevance Pistachio, Template_relevance Bkms_metabolic, Template_relevance Pistachio_ringbreaker, Template_relevance Reaxys, Template_relevance Reaxys_biocatalysis model, leveraging a vast database of chemical reactions to predict feasible synthetic routes.
One-Step Synthesis Focus: Specifically designed for one-step synthesis, it provides concise and direct routes for your target compounds, streamlining the synthesis process.
Accurate Predictions: Utilizing the extensive PISTACHIO, BKMS_METABOLIC, PISTACHIO_RINGBREAKER, REAXYS, REAXYS_BIOCATALYSIS database, our tool offers high-accuracy predictions, reflecting the latest in chemical research and data.
Strategy Settings
Precursor scoring | Relevance Heuristic |
---|---|
Min. plausibility | 0.01 |
Model | Template_relevance |
Template Set | Pistachio/Bkms_metabolic/Pistachio_ringbreaker/Reaxys/Reaxys_biocatalysis |
Top-N result to add to graph | 6 |
Feasible Synthetic Routes
Q1: What is the primary target of haloxyfop in plants?
A1: this compound specifically targets the Acetyl-CoA Carboxylase (ACCase) enzyme in susceptible plants. []
Q2: How does this compound affect ACCase activity?
A2: this compound inhibits ACCase activity, which is a crucial enzyme involved in fatty acid biosynthesis. [] This inhibition disrupts lipid production, ultimately leading to plant death. [, ]
Q3: What are the downstream effects of this compound-induced ACCase inhibition?
A3: ACCase inhibition leads to a cascade of effects, including:* Disruption of lipid synthesis, particularly affecting cell membrane formation. [, ]* Impaired respiration, primarily due to inhibition of the pyruvate dehydrogenase complex (PDC) and the α-ketoglutarate dehydrogenase complex (α-KGDC). []* Accumulation of amino acids within the plant cells. []
Q4: How does moisture stress influence the effectiveness of this compound?
A4: Moisture stress has been shown to reduce the efficacy of this compound. This is attributed to:
- Decreased foliar absorption of the herbicide. [, ]
- Inhibition of this compound translocation within the plant, affecting its movement to active sites. [, ]
Q5: Does moisture stress influence the metabolism of this compound in plants?
A5: Research suggests that while moisture stress significantly impacts this compound absorption and translocation, it does not appear to alter the herbicide's metabolism within the plants. [, ]
Q6: What is the molecular formula and weight of this compound?
A6: this compound has the molecular formula C15H11ClF3NO4 and a molecular weight of 373.7 g/mol.
Q7: Are there any known interactions between this compound and other herbicides?
A7: Yes, studies have shown both antagonistic and synergistic interactions. For instance:
- Mixing this compound with 2,4-D can reduce its efficacy, indicating antagonism. [, ]
- Combining this compound with chlorimuron or cloransulam can enhance weed control, suggesting synergy. []
Q8: How does the addition of adjuvants affect this compound's activity?
A8: Adjuvants can significantly influence this compound's effectiveness:
- Petroleum oil concentrate (POC) improves foliar absorption and translocation compared to soybean oil concentrate (SBOC), oxysorbic (20 POE), or no adjuvant. []
- Oxysorbic (20 POE) results in the lowest absorption of this compound among the tested adjuvants. []
Q9: Does relative humidity impact this compound efficacy?
A9: Yes, higher relative humidity (70%) generally leads to increased absorption and translocation of this compound in plants compared to lower relative humidity (30%). []
Disclaimer and Information on In-Vitro Research Products
Please be aware that all articles and product information presented on BenchChem are intended solely for informational purposes. The products available for purchase on BenchChem are specifically designed for in-vitro studies, which are conducted outside of living organisms. In-vitro studies, derived from the Latin term "in glass," involve experiments performed in controlled laboratory settings using cells or tissues. It is important to note that these products are not categorized as medicines or drugs, and they have not received approval from the FDA for the prevention, treatment, or cure of any medical condition, ailment, or disease. We must emphasize that any form of bodily introduction of these products into humans or animals is strictly prohibited by law. It is essential to adhere to these guidelines to ensure compliance with legal and ethical standards in research and experimentation.