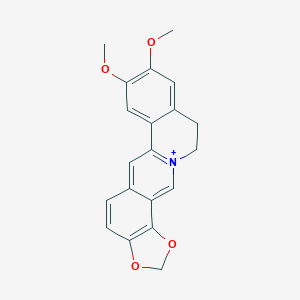
Epiberberine
Overview
Description
Epiberberine is a natural protoberberine alkaloid found in various plants, particularly in the rhizomes of Coptis species. It is known for its potential biological activities, including antimicrobial, anti-inflammatory, and enzyme inhibitory properties. This compound has garnered attention for its ability to inhibit rumen microbial urease, making it a promising candidate for reducing ammonia release in ruminant livestock .
Mechanism of Action
Target of Action
Epiberberine, a derivative of berberine, has been shown to have several cellular and molecular targets. It has been found to have a significant impact on metabolic disorders . It can inhibit toxins and bacteria, including Helicobacter pylori, protect the intestinal epithelial barrier from injury, and ameliorate liver injury .
Mode of Action
This compound interacts with its targets in a complex manner. It has been shown to have remarkable anti-inflammatory, antioxidant, antiapoptotic, and antiautophagic activity via the regulation of multiple signaling pathways, including AMP-activated protein kinase (AMPK), nuclear factor κB (NF-κB), mitogen-activated protein kinase silent information regulator 1 (SIRT-1), hypoxia-inducible factor 1α (HIF-1α), vascular endothelial growth factor phosphoinositide 3-kinase (PI3K), protein kinase B (Akt), janus kinase 2 (JAK-2), Ca 2+ channels, and endoplasmic reticulum stress .
Biochemical Pathways
This compound affects several biochemical pathways. It shows remarkable anti-inflammatory, antioxidant, antiapoptotic, and antiautophagic activity via the regulation of multiple signaling pathways, including AMP-activated protein kinase (AMPK), nuclear factor κB (NF-κB), mitogen-activated protein kinase silent information regulator 1 (SIRT-1), hypoxia-inducible factor 1α (HIF-1α), vascular endothelial growth factor phosphoinositide 3-kinase (PI3K), protein kinase B (Akt), janus kinase 2 (JAK-2), Ca 2+ channels, and endoplasmic reticulum stress .
Pharmacokinetics
The pharmacokinetics of this compound, like its parent compound berberine, involves the absorption, distribution, metabolism, and excretion (ADME) properties of the compound within a living organism that determine the safety and effectiveness of the drug .
Result of Action
The molecular and cellular effects of this compound’s action are significant. It has been shown to have potent neuroprotective effects, including antioxidative, antiapoptotic, and anti-ischemic . It also has a substantial impact on metabolic disorders, including the regulation of triglyceride (TG), total cholesterol (TC), low-density lipoprotein (LDL), high-density lipoprotein (HDL), homeostasis model assessment-insulin resistance (HOMA-IR), and fasting plasma glucose (FPG) .
Biochemical Analysis
Biochemical Properties
Epiberberine plays a crucial role in various biochemical reactions. It interacts with several enzymes, proteins, and other biomolecules. Notably, this compound has been shown to inhibit the activity of enzymes such as acetylcholinesterase and monoamine oxidase, which are involved in neurotransmitter regulation. Additionally, this compound interacts with proteins involved in inflammatory pathways, such as nuclear factor kappa B (NF-κB), thereby exerting anti-inflammatory effects .
Cellular Effects
This compound influences various cellular processes and functions. In adipocytes, this compound has been observed to reduce lipid accumulation by inhibiting cellular triglyceride synthesis . It also affects cell signaling pathways, including the AMP-activated protein kinase (AMPK) pathway, which plays a role in cellular energy homeostasis. Furthermore, this compound modulates gene expression related to inflammation and oxidative stress, thereby protecting cells from damage .
Molecular Mechanism
The molecular mechanism of this compound involves several pathways. This compound binds to and inhibits the activity of key enzymes, such as acetylcholinesterase, leading to increased levels of acetylcholine in the synaptic cleft. This action enhances cholinergic signaling, which is beneficial in neurodegenerative conditions. Additionally, this compound activates the AMPK pathway, promoting glucose uptake and lipid metabolism. It also inhibits the NF-κB pathway, reducing the expression of pro-inflammatory cytokines .
Dosage Effects in Animal Models
The effects of this compound vary with different dosages in animal models. At lower doses, this compound has been shown to improve cognitive function and reduce inflammation without significant adverse effects. At higher doses, this compound may exhibit toxic effects, including hepatotoxicity and nephrotoxicity. It is crucial to determine the optimal dosage that maximizes therapeutic benefits while minimizing potential risks .
Metabolic Pathways
This compound is involved in several metabolic pathways. It is metabolized primarily in the liver, where it undergoes phase I and phase II biotransformation. Enzymes such as cytochrome P450 (CYP450) play a significant role in its metabolism. This compound also affects metabolic flux by modulating the activity of key enzymes involved in glucose and lipid metabolism, thereby influencing metabolite levels .
Transport and Distribution
This compound is transported and distributed within cells and tissues through various mechanisms. It is rapidly absorbed in the gastrointestinal tract and distributed to tissues such as the liver, kidneys, and brain. Transporters such as P-glycoprotein (P-gp) and organic cation transporters (OCTs) facilitate its cellular uptake and distribution. This compound’s localization in specific tissues contributes to its therapeutic effects .
Subcellular Localization
This compound exhibits specific subcellular localization, which influences its activity and function. It is predominantly localized in the cytoplasm and mitochondria, where it exerts its antioxidant and metabolic effects. This compound’s targeting to mitochondria is facilitated by its lipophilic nature, allowing it to interact with mitochondrial membranes and modulate mitochondrial function. Additionally, this compound may undergo post-translational modifications that direct it to specific cellular compartments .
Preparation Methods
Synthetic Routes and Reaction Conditions: Epiberberine can be synthesized through various chemical routes. One common method involves the extraction of the compound from plant sources such as Coptis rhizomes. The extraction process typically includes steps like drying, grinding, and solvent extraction using ethanol or methanol. The crude extract is then purified using chromatographic techniques to isolate this compound.
Industrial Production Methods: Industrial production of this compound involves large-scale extraction from plant materials. The process includes:
Harvesting and Drying: Collecting the plant material and drying it to reduce moisture content.
Grinding: Grinding the dried material into a fine powder.
Solvent Extraction: Using solvents like ethanol or methanol to extract the alkaloids.
Purification: Employing chromatographic methods to purify the extract and isolate this compound.
Chemical Reactions Analysis
Types of Reactions: Epiberberine undergoes various chemical reactions, including:
Oxidation: this compound can be oxidized to form different derivatives.
Reduction: Reduction reactions can modify the structure of this compound, leading to the formation of reduced derivatives.
Substitution: Substitution reactions can introduce new functional groups into the this compound molecule.
Common Reagents and Conditions:
Oxidizing Agents: Hydrogen peroxide, potassium permanganate.
Reducing Agents: Sodium borohydride, lithium aluminum hydride.
Substitution Reagents: Halogens, alkylating agents.
Major Products: The major products formed from these reactions include various this compound derivatives with modified biological activities.
Scientific Research Applications
Epiberberine has a wide range of scientific research applications:
Chemistry: Used as a precursor for synthesizing other bioactive compounds.
Biology: Studied for its antimicrobial and enzyme inhibitory properties.
Medicine: Investigated for its potential therapeutic effects, including anti-inflammatory and anticancer activities.
Industry: Utilized as a natural feed additive to reduce ammonia release in ruminant livestock.
Comparison with Similar Compounds
Epiberberine is structurally similar to other protoberberine alkaloids such as berberine, palmatine, and coptisine. it exhibits unique properties:
Berberine: Known for its broad-spectrum antimicrobial and anti-inflammatory activities.
Palmatine: Exhibits antimicrobial and anti-inflammatory properties but with different potency.
Coptisine: Similar to berberine but with distinct pharmacological effects.
This compound stands out due to its superior inhibition of rumen microbial urease, making it a valuable compound for reducing ammonia release in ruminant livestock .
Properties
IUPAC Name |
16,17-dimethoxy-5,7-dioxa-1-azoniapentacyclo[11.8.0.03,11.04,8.014,19]henicosa-1(13),2,4(8),9,11,14,16,18-octaene | |
---|---|---|
Source | PubChem | |
URL | https://pubchem.ncbi.nlm.nih.gov | |
Description | Data deposited in or computed by PubChem | |
InChI |
InChI=1S/C20H18NO4/c1-22-18-8-13-5-6-21-10-15-12(3-4-17-20(15)25-11-24-17)7-16(21)14(13)9-19(18)23-2/h3-4,7-10H,5-6,11H2,1-2H3/q+1 | |
Source | PubChem | |
URL | https://pubchem.ncbi.nlm.nih.gov | |
Description | Data deposited in or computed by PubChem | |
InChI Key |
FPJQGFLUORYYPE-UHFFFAOYSA-N | |
Source | PubChem | |
URL | https://pubchem.ncbi.nlm.nih.gov | |
Description | Data deposited in or computed by PubChem | |
Canonical SMILES |
COC1=C(C=C2C(=C1)CC[N+]3=C2C=C4C=CC5=C(C4=C3)OCO5)OC | |
Source | PubChem | |
URL | https://pubchem.ncbi.nlm.nih.gov | |
Description | Data deposited in or computed by PubChem | |
Molecular Formula |
C20H18NO4+ | |
Source | PubChem | |
URL | https://pubchem.ncbi.nlm.nih.gov | |
Description | Data deposited in or computed by PubChem | |
DSSTOX Substance ID |
DTXSID90218854 | |
Record name | Epiberberine | |
Source | EPA DSSTox | |
URL | https://comptox.epa.gov/dashboard/DTXSID90218854 | |
Description | DSSTox provides a high quality public chemistry resource for supporting improved predictive toxicology. | |
Molecular Weight |
336.4 g/mol | |
Source | PubChem | |
URL | https://pubchem.ncbi.nlm.nih.gov | |
Description | Data deposited in or computed by PubChem | |
CAS No. |
6873-09-2 | |
Record name | Epiberberine | |
Source | CAS Common Chemistry | |
URL | https://commonchemistry.cas.org/detail?cas_rn=6873-09-2 | |
Description | CAS Common Chemistry is an open community resource for accessing chemical information. Nearly 500,000 chemical substances from CAS REGISTRY cover areas of community interest, including common and frequently regulated chemicals, and those relevant to high school and undergraduate chemistry classes. This chemical information, curated by our expert scientists, is provided in alignment with our mission as a division of the American Chemical Society. | |
Explanation | The data from CAS Common Chemistry is provided under a CC-BY-NC 4.0 license, unless otherwise stated. | |
Record name | Epiberberine | |
Source | ChemIDplus | |
URL | https://pubchem.ncbi.nlm.nih.gov/substance/?source=chemidplus&sourceid=0006873092 | |
Description | ChemIDplus is a free, web search system that provides access to the structure and nomenclature authority files used for the identification of chemical substances cited in National Library of Medicine (NLM) databases, including the TOXNET system. | |
Record name | Epiberberine | |
Source | EPA DSSTox | |
URL | https://comptox.epa.gov/dashboard/DTXSID90218854 | |
Description | DSSTox provides a high quality public chemistry resource for supporting improved predictive toxicology. | |
Record name | EPIBERBERINE ION | |
Source | FDA Global Substance Registration System (GSRS) | |
URL | https://gsrs.ncats.nih.gov/ginas/app/beta/substances/VWP9N35AYS | |
Description | The FDA Global Substance Registration System (GSRS) enables the efficient and accurate exchange of information on what substances are in regulated products. Instead of relying on names, which vary across regulatory domains, countries, and regions, the GSRS knowledge base makes it possible for substances to be defined by standardized, scientific descriptions. | |
Explanation | Unless otherwise noted, the contents of the FDA website (www.fda.gov), both text and graphics, are not copyrighted. They are in the public domain and may be republished, reprinted and otherwise used freely by anyone without the need to obtain permission from FDA. Credit to the U.S. Food and Drug Administration as the source is appreciated but not required. | |
Retrosynthesis Analysis
AI-Powered Synthesis Planning: Our tool employs the Template_relevance Pistachio, Template_relevance Bkms_metabolic, Template_relevance Pistachio_ringbreaker, Template_relevance Reaxys, Template_relevance Reaxys_biocatalysis model, leveraging a vast database of chemical reactions to predict feasible synthetic routes.
One-Step Synthesis Focus: Specifically designed for one-step synthesis, it provides concise and direct routes for your target compounds, streamlining the synthesis process.
Accurate Predictions: Utilizing the extensive PISTACHIO, BKMS_METABOLIC, PISTACHIO_RINGBREAKER, REAXYS, REAXYS_BIOCATALYSIS database, our tool offers high-accuracy predictions, reflecting the latest in chemical research and data.
Strategy Settings
Precursor scoring | Relevance Heuristic |
---|---|
Min. plausibility | 0.01 |
Model | Template_relevance |
Template Set | Pistachio/Bkms_metabolic/Pistachio_ringbreaker/Reaxys/Reaxys_biocatalysis |
Top-N result to add to graph | 6 |
Feasible Synthetic Routes
Q1: What is the primary biological target of epiberberine?
A1: this compound demonstrates a strong binding affinity for the human telomeric G-quadruplex (HTG) DNA structure, specifically the hybrid-2 form prevalent in potassium-rich environments. [] This interaction stabilizes the HTG and can inhibit telomerase, an enzyme implicated in cancer cell immortality. [, ]
Q2: How does this compound binding affect the human telomeric G-quadruplex structure?
A2: this compound induces a significant conformational change in the HTG upon binding. It primarily targets the 5' end, recruiting the flanking adenine to form a unique \"quasi-triad\" that intercalates with the G-tetrad and surrounding capping structures. This interaction leads to a well-defined, multi-layer binding pocket exclusive to the hybrid-2 HTG conformation. [, , ]
Q3: What are the downstream consequences of this compound's interaction with the HTG?
A3: By stabilizing the HTG, this compound can inhibit the activity of telomerase. [, , ] Telomerase is responsible for maintaining telomere length, and its inhibition can lead to telomere shortening and ultimately, cancer cell death.
Q4: Does this compound interact with any other biological targets?
A4: Yes, this compound has been shown to inhibit certain cytochrome P450 (CYP450) isoforms, specifically CYP2C9 and CYP2D6, in human liver microsomes. [] It also interacts with human organic cation transporters (OCTs), acting as both an inhibitor and substrate for OCT1, OCT2, and OCT3. []
Q5: What is the significance of this compound’s interaction with OCTs?
A5: OCTs play a crucial role in drug absorption, distribution, and elimination. this compound’s interaction with OCTs suggests a potential for drug-drug interactions and highlights the importance of considering these interactions in clinical settings. []
Q6: What is the molecular formula and weight of this compound?
A6: The molecular formula of this compound is C20H17NO4, and its molecular weight is 335.35 g/mol.
Q7: Are there any spectroscopic data available for this compound?
A7: While the provided research excerpts don't contain specific spectroscopic data, they commonly utilize techniques like High-Performance Liquid Chromatography (HPLC) [, , , , , ], Liquid Chromatography-Mass Spectrometry (LC-MS/MS) [, , , ], and Nuclear Magnetic Resonance (NMR) spectroscopy [, ] for identification and characterization of this compound.
Q8: How do structural modifications of this compound affect its biological activity?
A8: Research suggests that the 9,10-methylenedioxy and 2,3-dimethoxy groups in this compound's structure might play a role in its interaction with DNA. [] Comparing this compound's activity with other protoberberine alkaloids reveals that subtle structural differences significantly influence their binding affinities to the HTG and other targets like CYP450 enzymes. [, ] For instance, this compound exhibits a stronger inhibitory effect on CYP2D6 compared to berberine, jatrorrhizine, and palmatine. []
Q9: How is this compound distributed in the body?
A11: Studies in rats indicate that this compound is widely distributed in various tissues, including the liver, heart, kidney, and others. []
Q10: How is this compound metabolized?
A12: this compound undergoes extensive metabolism, primarily in the liver. [, ] Identified metabolic pathways include demethylation, hydroxylation, sulfation, and glucuronidation. []
Q11: How is this compound excreted?
A13: Following oral administration, this compound is primarily excreted through bile and feces, with a significant portion also eliminated via the kidneys. []
Q12: Are there any known drug-drug interactions with this compound?
A14: Yes, this compound's inhibition of CYP2C9 and CYP2D6 in human liver microsomes indicates a potential for interactions with drugs metabolized by these enzymes. [] Furthermore, its interaction with OCTs could also contribute to drug-drug interactions. []
Q13: What in vitro models have been used to study the biological activity of this compound?
A15: this compound's activity has been investigated in various in vitro models, including cell-based assays using human cancer cell lines like NCI-N87 (gastric cancer) and Caco-2 (colon cancer), demonstrating inhibitory effects on cancer cell proliferation. [] It has also been studied in enzyme assays targeting BACE1, AChE, BChE, and others to evaluate its anti-Alzheimer's and antioxidant potential. []
Q14: What in vivo models have been used to study the biological activity of this compound?
A16: this compound's effects have been explored in rat models to assess its pharmacokinetic properties, tissue distribution, and potential against scopolamine-induced amnesia. [, , , ] Zebrafish models have also been employed to investigate its metabolic pathways. []
Q15: What analytical methods are commonly used for the detection and quantification of this compound?
A17: The most frequently employed techniques include High-Performance Liquid Chromatography (HPLC) [, , , , , ] coupled with various detectors like Diode Array Detection (DAD) [, ] and Mass Spectrometry (MS) [, , ]. Liquid Chromatography-Tandem Mass Spectrometry (LC-MS/MS) is particularly valuable for its sensitivity and specificity in quantifying this compound in biological samples. [, , , , ]
Disclaimer and Information on In-Vitro Research Products
Please be aware that all articles and product information presented on BenchChem are intended solely for informational purposes. The products available for purchase on BenchChem are specifically designed for in-vitro studies, which are conducted outside of living organisms. In-vitro studies, derived from the Latin term "in glass," involve experiments performed in controlled laboratory settings using cells or tissues. It is important to note that these products are not categorized as medicines or drugs, and they have not received approval from the FDA for the prevention, treatment, or cure of any medical condition, ailment, or disease. We must emphasize that any form of bodily introduction of these products into humans or animals is strictly prohibited by law. It is essential to adhere to these guidelines to ensure compliance with legal and ethical standards in research and experimentation.