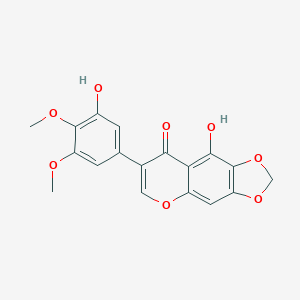
Dichotomitin
Overview
Description
Dichotomitin is an isoflavonoid compound with the molecular formula C18H14O8. It is isolated from the rhizomes of Belamcanda chinensis (L.) DC, a plant commonly used in traditional Chinese medicine . This compound is known for its various biological activities and potential therapeutic applications.
Preparation Methods
Synthetic Routes and Reaction Conditions
Dichotomitin can be synthesized through several chemical reactions involving the appropriate starting materials and reagents. The synthetic routes typically involve the use of methoxy and hydroxy-substituted benzene derivatives, followed by cyclization reactions to form the isoflavonoid structure .
Industrial Production Methods
Industrial production of this compound involves the extraction of the compound from the rhizomes of Belamcanda chinensis. The extraction process includes drying and grinding the plant material, followed by solvent extraction using organic solvents such as methanol or ethanol. The extract is then purified using chromatographic techniques to isolate this compound .
Chemical Reactions Analysis
Types of Reactions
Dichotomitin undergoes various chemical reactions, including:
Oxidation: this compound can be oxidized to form quinones and other oxidized derivatives.
Reduction: Reduction reactions can convert this compound into its reduced forms, such as dihydro derivatives.
Substitution: Substitution reactions involve the replacement of functional groups on the benzene ring with other substituents.
Common Reagents and Conditions
Oxidation: Common oxidizing agents include potassium permanganate (KMnO4) and chromium trioxide (CrO3).
Reduction: Reducing agents such as sodium borohydride (NaBH4) and lithium aluminum hydride (LiAlH4) are used.
Major Products Formed
The major products formed from these reactions include various oxidized, reduced, and substituted derivatives of this compound, which can have different biological activities and properties .
Scientific Research Applications
Dichotomitin has a wide range of scientific research applications, including:
Chemistry: Used as a reference compound in the study of isoflavonoids and their chemical properties.
Biology: Investigated for its potential anti-inflammatory, antioxidant, and anticancer activities.
Medicine: Explored for its therapeutic potential in treating diseases such as cancer, inflammation, and oxidative stress-related disorders.
Industry: Utilized in the development of natural product-based pharmaceuticals and nutraceuticals.
Mechanism of Action
Dichotomitin exerts its effects through various molecular targets and pathways. It is known to interact with enzymes and receptors involved in inflammation, oxidative stress, and cell proliferation. The compound can inhibit the activity of pro-inflammatory enzymes, scavenge free radicals, and induce apoptosis in cancer cells .
Comparison with Similar Compounds
Similar Compounds
Tectoridin: Another isoflavonoid with similar anti-inflammatory and antioxidant properties.
Irigenin: Known for its anticancer and hepatoprotective activities.
Irisflorentine: Exhibits anti-inflammatory and antioxidant effects.
Uniqueness of Dichotomitin
This compound is unique due to its specific chemical structure, which includes methoxy and hydroxy substituents on the benzene ring, and its ability to form various derivatives through chemical reactions. This structural uniqueness contributes to its diverse biological activities and potential therapeutic applications .
Properties
IUPAC Name |
9-hydroxy-7-(3-hydroxy-4,5-dimethoxyphenyl)-[1,3]dioxolo[4,5-g]chromen-8-one | |
---|---|---|
Source | PubChem | |
URL | https://pubchem.ncbi.nlm.nih.gov | |
Description | Data deposited in or computed by PubChem | |
InChI |
InChI=1S/C18H14O8/c1-22-12-4-8(3-10(19)17(12)23-2)9-6-24-11-5-13-18(26-7-25-13)16(21)14(11)15(9)20/h3-6,19,21H,7H2,1-2H3 | |
Source | PubChem | |
URL | https://pubchem.ncbi.nlm.nih.gov | |
Description | Data deposited in or computed by PubChem | |
InChI Key |
PFFOGGCBLWTCPM-UHFFFAOYSA-N | |
Source | PubChem | |
URL | https://pubchem.ncbi.nlm.nih.gov | |
Description | Data deposited in or computed by PubChem | |
Canonical SMILES |
COC1=CC(=CC(=C1OC)O)C2=COC3=CC4=C(C(=C3C2=O)O)OCO4 | |
Source | PubChem | |
URL | https://pubchem.ncbi.nlm.nih.gov | |
Description | Data deposited in or computed by PubChem | |
Molecular Formula |
C18H14O8 | |
Source | PubChem | |
URL | https://pubchem.ncbi.nlm.nih.gov | |
Description | Data deposited in or computed by PubChem | |
DSSTOX Substance ID |
DTXSID10237087 | |
Record name | Dichotomitin | |
Source | EPA DSSTox | |
URL | https://comptox.epa.gov/dashboard/DTXSID10237087 | |
Description | DSSTox provides a high quality public chemistry resource for supporting improved predictive toxicology. | |
Molecular Weight |
358.3 g/mol | |
Source | PubChem | |
URL | https://pubchem.ncbi.nlm.nih.gov | |
Description | Data deposited in or computed by PubChem | |
CAS No. |
88509-91-5 | |
Record name | Dichotomitin | |
Source | ChemIDplus | |
URL | https://pubchem.ncbi.nlm.nih.gov/substance/?source=chemidplus&sourceid=0088509915 | |
Description | ChemIDplus is a free, web search system that provides access to the structure and nomenclature authority files used for the identification of chemical substances cited in National Library of Medicine (NLM) databases, including the TOXNET system. | |
Record name | Dichotomitin | |
Source | EPA DSSTox | |
URL | https://comptox.epa.gov/dashboard/DTXSID10237087 | |
Description | DSSTox provides a high quality public chemistry resource for supporting improved predictive toxicology. | |
Retrosynthesis Analysis
AI-Powered Synthesis Planning: Our tool employs the Template_relevance Pistachio, Template_relevance Bkms_metabolic, Template_relevance Pistachio_ringbreaker, Template_relevance Reaxys, Template_relevance Reaxys_biocatalysis model, leveraging a vast database of chemical reactions to predict feasible synthetic routes.
One-Step Synthesis Focus: Specifically designed for one-step synthesis, it provides concise and direct routes for your target compounds, streamlining the synthesis process.
Accurate Predictions: Utilizing the extensive PISTACHIO, BKMS_METABOLIC, PISTACHIO_RINGBREAKER, REAXYS, REAXYS_BIOCATALYSIS database, our tool offers high-accuracy predictions, reflecting the latest in chemical research and data.
Strategy Settings
Precursor scoring | Relevance Heuristic |
---|---|
Min. plausibility | 0.01 |
Model | Template_relevance |
Template Set | Pistachio/Bkms_metabolic/Pistachio_ringbreaker/Reaxys/Reaxys_biocatalysis |
Top-N result to add to graph | 6 |
Feasible Synthetic Routes
Q1: What is dichotomitin and where is it found?
A1: this compound is an isoflavone primarily found in the rhizomes of Belamcanda chinensis, also known as blackberry lily. It was first isolated from Iris dichotoma Pall. []. This plant is used in traditional Chinese medicine.
Q2: What are the main metabolic pathways of this compound?
A2: this compound undergoes various metabolic transformations, including demethylation, hydroxylation, glucuronidation, and sulfation. One major metabolic pathway is the opening of the 1,3-benzodioxole ring [].
Q3: Which cytochrome P450 enzymes are involved in this compound metabolism?
A3: In vitro studies using human recombinant enzymes revealed that CYP1A2, 2C19, and 2D6 contribute to the formation of the metabolite 3',5,6,7-tetrahydroxy-4',5'-dimethoxy isoflavone (M6). Interestingly, CYP1A2 exclusively catalyzes the formation of another major metabolite, 8-hydroxy-dichotomitin (M8) [].
Q4: Can you describe the structural characteristics of this compound?
A4: this compound is a 5,3′-dihydroxy-4′,5′-dimethoxy-6,7-methylenedioxyisoflavone []. While the provided research papers don't explicitly state the molecular formula and weight, this information can be deduced from the chemical structure.
Q5: How does the content of this compound vary within Belamcanda chinensis?
A5: this compound content varies significantly within different parts of the Belamcanda chinensis plant. The eldest parts of the rhizome, directly originating from the seedling, generally exhibit higher concentrations of this compound and other flavonoids compared to the younger parts [].
Q6: Are there any analytical methods available to quantify this compound?
A7: Yes, high-performance liquid chromatography (HPLC) coupled with various detection methods, particularly ultraviolet (UV) detection, is widely employed for quantifying this compound and other isoflavones in Belamcanda chinensis extracts [, , , ].
Q7: What is the significance of understanding the metabolic fate of this compound?
A8: Characterizing the metabolic pathways and enzymes involved in this compound breakdown is crucial for understanding its disposition within the body. This knowledge is essential for assessing its potential efficacy and safety as a therapeutic agent [].
Q8: How do the levels of this compound compare in fresh and dried Belamcanda chinensis and other Iris species?
A9: The levels of this compound and other isoflavones can differ between fresh and dried forms of Belamcanda chinensis and other Iris species. For instance, iridin is the most abundant isoflavone in both fresh and dried Belamcanda chinensis, while irisflorentin dominates in Iris germanica. Interestingly, these isoflavones are barely detectable in Iris japonica []. This highlights the variability in phytochemical profiles among different Iris species.
Disclaimer and Information on In-Vitro Research Products
Please be aware that all articles and product information presented on BenchChem are intended solely for informational purposes. The products available for purchase on BenchChem are specifically designed for in-vitro studies, which are conducted outside of living organisms. In-vitro studies, derived from the Latin term "in glass," involve experiments performed in controlled laboratory settings using cells or tissues. It is important to note that these products are not categorized as medicines or drugs, and they have not received approval from the FDA for the prevention, treatment, or cure of any medical condition, ailment, or disease. We must emphasize that any form of bodily introduction of these products into humans or animals is strictly prohibited by law. It is essential to adhere to these guidelines to ensure compliance with legal and ethical standards in research and experimentation.