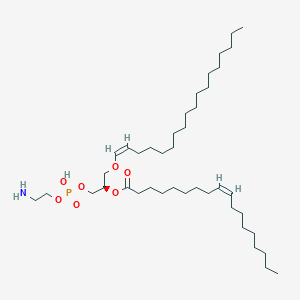
1-(1Z-octadecenyl)-2-(9Z-octadecenoyl)-sn-glycero-3-phosphoethanolamine
Overview
Description
1-(1Z-octadecenyl)-2-(9Z-octadecenoyl)-sn-glycero-3-phosphoethanolamine (systematic name: PE(P-18:0/18:1(9Z))) is a plasmalogen-type glycerophosphoethanolamine (GPEtn) characterized by an ether-linked 1Z-octadecenyl chain at the sn-1 position and an ester-linked 9Z-octadecenoyl (oleoyl) chain at the sn-2 position . Its molecular formula is C41H80NO7P, with a molecular weight of 730.05 g/mol . Structurally, it belongs to the GP02 lipid class (glycerophosphoethanolamines) and is distinguished by its ether bond at sn-1, a hallmark of plasmalogens, which confers unique biophysical properties such as enhanced membrane fluidity and resistance to oxidative stress .
This compound is biosynthetically linked to the glycerophospho-ethanolamine pathway and is detected in negative ionization mode during lipidomic analyses . In infection models, it is identified as a significantly upregulated lipid metabolite, peaking at 81 hours post-infection, suggesting a role in host-pathogen interactions or inflammatory responses .
Biological Activity
1-(1Z-octadecenyl)-2-(9Z-octadecenoyl)-sn-glycero-3-phosphoethanolamine, commonly referred to as PE(18:1/18:1), is a phospholipid that plays a significant role in cellular membranes and various biological processes. This compound is structurally characterized by the presence of two unsaturated fatty acid chains, which contribute to its fluidity and functionality in biological systems. Its biological activity encompasses a wide range of effects, including its involvement in membrane dynamics, signaling pathways, and metabolic processes.
Membrane Dynamics
Phospholipids like PE(18:1/18:1) are crucial components of cellular membranes. They contribute to membrane fluidity and flexibility, which are essential for various cellular functions such as:
- Vesicle Formation : PE is involved in the formation of lipid bilayers and vesicles, facilitating intracellular transport.
- Protein Functionality : The presence of unsaturated fatty acids enhances the functionality of membrane proteins, affecting their conformation and activity.
Signaling Pathways
PE(18:1/18:1) is implicated in several signaling pathways:
- Cell Proliferation and Survival : Studies indicate that phosphatidylethanolamine can influence pathways related to cell growth and apoptosis. For instance, it has been shown to modulate the activity of certain kinases involved in cell survival .
- Inflammatory Response : This compound may play a role in modulating inflammatory responses by influencing the production of signaling molecules such as cytokines .
Metabolic Processes
Recent research has highlighted the metabolic roles of PE(18:1/18:1):
- Lipid Metabolism : It is involved in the metabolism of lipids, contributing to the synthesis and degradation of other lipid species .
- Antioxidant Activity : There are indications that phospholipids can exhibit antioxidant properties, helping to mitigate oxidative stress within cells .
Case Studies
Several studies have explored the biological activity of PE(18:1/18:1):
- Prenatal Exposure Study :
-
Cell Viability and Metabolic Activity :
- In a study utilizing a cell painting assay combined with metabolomics, researchers evaluated the effects of various nanoparticles on lung epithelial cells. The results indicated that exposure to certain concentrations of nanoparticles led to reduced metabolic activity and increased reactive oxygen species (ROS) production, highlighting the potential protective role of phospholipids like PE against oxidative stress .
Data Table
Study | Focus | Key Findings |
---|---|---|
Prenatal Exposure Study | Impact on metabolome | Altered lipid profiles linked to developmental outcomes |
Cell Viability Study | Effects on lung cells | Reduced metabolic activity at high nanoparticle concentrations |
Scientific Research Applications
Molecular Formula
- Chemical Formula : C₄₁H₈₀N₀₇P
- Molecular Weight : 729.57 g/mol
Structural Characteristics
- Contains two unsaturated fatty acyl chains (oleic acid derivatives).
- Exhibits zwitterionic properties, contributing to its amphiphilic nature.
Cell Membrane Studies
PE 18:1/18:1 is integral to the composition of cell membranes, influencing fluidity and permeability. Research has shown that variations in phospholipid composition can affect membrane dynamics, which is crucial for understanding cellular processes such as:
- Membrane fusion : Important in neurotransmitter release and viral entry into cells.
- Signal transduction : Acts as a precursor for signaling molecules.
Lipid Metabolism Research
Recent studies have highlighted the role of PE 18:1/18:1 in lipid metabolism, particularly in the context of:
- Hepatocellular carcinoma : A study indicated that circular RNA CircLARP2 regulates lipid metabolism and metastasis in liver cancer, with PE 18:1/18:1 being a significant metabolite involved in these pathways .
Study Focus | Findings |
---|---|
Hepatocellular Carcinoma | Regulation of lipid metabolism by CircLARP2 involving PE 18:1/18:1 |
Drug Delivery Systems
Due to its biocompatibility and ability to form lipid bilayers, PE 18:1/18:1 is being explored for use in:
- Nanoparticle formulations : Enhancing drug solubility and targeting capabilities.
- Vaccine adjuvants : Improving immune responses through better antigen presentation.
Neurobiology
PE 18:1/18:1 is implicated in neuronal function and health. Studies suggest that alterations in phospholipid composition can affect:
- Neurotransmitter release : Modulating synaptic efficacy.
- Neurodegenerative diseases : Understanding how lipid dysregulation contributes to conditions like Alzheimer's disease.
Case Study 1: Impact on Cancer Metabolism
In a comprehensive study analyzing the metabolomic profiles of hepatocellular carcinoma patients, PE 18:1/18:1 was identified as a key metabolite associated with tumor progression. The study utilized mass spectrometry techniques to quantify lipid levels and correlate them with clinical outcomes, revealing potential therapeutic targets .
Case Study 2: Membrane Dynamics in Neurons
Research conducted on neuronal cell lines demonstrated that the incorporation of PE 18:1/18:1 into membranes enhanced fluidity, which facilitated increased neurotransmitter release during synaptic activity. This finding underscores the importance of specific phospholipids in maintaining neuronal health and function.
Q & A
Basic Research Questions
Q. How can the structural identity of 1-(1Z-octadecenyl)-2-(9Z-octadecenoyl)-sn-glycero-3-phosphoethanolamine be validated in lipidomics workflows?
Methodological Answer:
- Mass Spectrometry (MS): Use high-resolution tandem MS (HR-MS/MS) to confirm molecular weight (exact mass: ~745.56 g/mol) and fragmentation patterns. The sn-1 alkyl chain (1Z-octadecenyl) and sn-2 acyl chain (9Z-octadecenoyl) produce distinct ions (e.g., m/z 239 for oleoyl fragments) .
- NMR Spectroscopy: H and C NMR can resolve double-bond geometry (Z-configuration) via coupling constants (J = 10–12 Hz for cis double bonds) and carbonyl carbon shifts (~172–174 ppm) .
- Chromatography: Reverse-phase HPLC with a C18 column and evaporative light scattering detection (ELSD) separates regioisomers based on acyl/alkyl chain positions .
Q. What experimental strategies are used to synthesize this compound?
Methodological Answer:
- Enzymatic Synthesis: Use phospholipase D (PLD) to transphosphatidylate sn-3-phosphocholine derivatives, replacing the headgroup with ethanolamine .
- Chemical Synthesis:
- Purification: Silica gel chromatography with chloroform/methanol/ammonium hydroxide gradients removes unreacted precursors .
Q. How do storage conditions impact the stability of this ether-phospholipid?
Methodological Answer:
- Oxidation Prevention: Store under argon at −80°C in amber vials. Add 0.01% butylated hydroxytoluene (BHT) to inhibit radical-mediated oxidation of unsaturated bonds .
- Hydrolysis Mitigation: Avoid aqueous buffers with pH > 8.0 to prevent ester bond cleavage. Lyophilization is recommended for long-term storage .
Advanced Research Questions
Q. What role does the 1Z-octadecenyl ether moiety play in membrane dynamics compared to acyl-linked analogs?
Methodological Answer:
- Bilayer Fluidity: Use fluorescence anisotropy with DPH probes to compare membrane rigidity. Ether linkages reduce phase transition temperatures by ~5°C versus acyl analogs due to reduced van der Waals interactions .
- Lateral Diffusion: Employ FRAP (fluorescence recovery after photobleaching) with BODIPY-labeled analogs. Ether lipids exhibit 20–30% faster diffusion in lipid rafts .
- Protein Interactions: Surface plasmon resonance (SPR) reveals higher binding affinity (~2-fold) of ether-linked lipids to transmembrane receptors like TLR4 .
Q. How can conflicting data on this lipid’s role in apoptosis be resolved?
Methodological Answer:
- Controlled Model Systems: Compare apoptosis in ether lipid-deficient vs. wild-type cells (e.g., RAW 264.7 macrophages) using CRISPR-Cas9 knockout of AGPS (alkylglycerone phosphate synthase) .
- Metabolomic Profiling: LC-MS/MS quantifies downstream mediators (e.g., platelet-activating factor, PAF) to distinguish pro-apoptotic vs. anti-apoptotic pathways .
- Dose-Dependent Assays: Titrate the lipid (0.1–100 µM) in primary hepatocytes. Low doses (<10 µM) may activate survival kinases (e.g., Akt), while high doses induce mitochondrial permeabilization .
Q. What advanced analytical techniques differentiate isomeric contaminants in synthesized batches?
Methodological Answer:
- Ion Mobility-MS: Resolves sn-1/sn-2 regioisomers via collision cross-section (CCS) differences (ΔCCS > 2.5 Ų) .
- Chiral Chromatography: Use a CHIRALPAK IG-U column with hexane/isopropanol (95:5) to separate enantiomers (Rf difference = 0.15) .
- Isotope-Labeling: Synthesize C-labeled standards at the glycerol backbone to track positional integrity via C-H HSQC NMR .
Q. How does this lipid interact with polyunsaturated fatty acid (PUFA)-containing phospholipids in mixed bilayers?
Methodological Answer:
- Molecular Dynamics (MD) Simulations: GROMACS simulations with the Slipids forcefield show ether lipids reduce bilayer thickness by 3–4 Å when mixed with DHA-containing PE .
- DSC (Differential Scanning Calorimetry): Thermograms reveal co-crystallization with 18:0–22:6 PE, lowering melting enthalpy (ΔH) by 15% .
- Langmuir Trough Assays: Measure π-A isotherms; ether lipids increase monolayer compressibility (ΔA = 8 Ų/molecule) in PUFA-rich phases .
Q. Methodological Notes for Experimental Design
- Sample Preparation: For cell studies, incorporate the lipid into liposomes (e.g., 10 mol% in DOPC) using extrusion (100 nm pores) to ensure uniform size .
- Data Normalization: In lipidomics, normalize peak areas to internal standards (e.g., PE 14:0/14:0) to correct for ion suppression .
- Contradiction Resolution: Replicate conflicting studies under identical conditions (e.g., pH, temperature, cell type) and apply multivariate analysis (PCA) to identify confounding variables .
Comparison with Similar Compounds
Structural Analogues
Plasmalogens with Different Head Groups
- 1-(1Z-octadecenyl)-2-(9Z-octadecenoyl)-sn-glycero-3-phosphocholine (PC(P-18:0/18:1)) Head Group: Phosphocholine (PC) instead of phosphoethanolamine (PE). Function: PC plasmalogens are more abundant in cellular membranes and play roles in lipid raft formation and signaling. Analytical Differentiation: Detected in positive ionization mode due to the quaternary ammonium group in PC, unlike PE derivatives detected in negative mode . Molecular Weight: 772.15 g/mol (vs. 730.05 g/mol for PE) .
Ether-Linked Phosphoethanolamines with Varied Chains
- 1-Hexadecyl-2-(9Z-octadecenoyl)-sn-glycero-3-phosphoethanolamine (PE(16:0e/18:1)) Chain Differences: sn-1 alkyl chain is fully saturated (16:0e vs. 18:0p). Biological Impact: Shorter alkyl chains reduce membrane stability compared to longer chains in the target compound . Quantitative Detection: Used as an internal standard in lipidomics, with calibration curves spanning 100–2,500 ng/mL .
Functional Analogues
Diacyl-Phosphoethanolamines
- 1-Palmitoyl-2-oleoyl-sn-glycero-3-phosphoethanolamine (PE(16:0/18:1)) Bond Type: Ester bonds at both sn-1 and sn-2 positions. Stability: More susceptible to hydrolysis by phospholipases compared to ether-linked plasmalogens . Role: Predominantly involved in membrane asymmetry and apoptosis signaling .
Lysophosphatidylethanolamines (LPEs)
- 1-(9Z-octadecenoyl)-sn-glycero-3-phosphoethanolamine (LPE(18:1)) Structure: Single acyl chain at sn-1, lacking the ether-linked alkyl group. Function: LPEs act as signaling molecules in inflammation and lysosomal membrane disruption . Detection: Lower molecular weight (479.30 g/mol) compared to the target compound .
Analytical and Metabolic Comparisons
Quantification in Lipidomics
- Calibration Standards : The compound is quantified alongside PC(16:0e/18:1), PC(18:0p/18:1), and PE(16:0/18:1) using LC-MS/MS, with linear ranges of 100–2,500 ng/mL .
- Ionization Efficiency: Higher sensitivity in negative mode due to the phosphate-ethanolamine group, unlike PC analogues requiring positive mode .
Metabolic Pathways
- Biosynthesis : Synthesized via the peroxisomal plasmalogen pathway, contrasting with diacyl-PEs produced in the endoplasmic reticulum .
- Degradation : Susceptible to plasmalogen-selective phospholipase A2 (PLA2), releasing free fatty acids and lysoplasmalogens .
Data Tables
Table 1: Structural Comparison of Selected Phosphoethanolamines
Compound Name | Molecular Formula | Molecular Weight (g/mol) | Bond Type (sn-1) | Key Biological Role |
---|---|---|---|---|
PE(P-18:0/18:1(9Z)) (Target Compound) | C41H80NO7P | 730.05 | Ether | Membrane stability, signaling |
PE(16:0/18:1) | C39H76NO8P | 718.00 | Ester | Apoptosis, membrane asymmetry |
LPE(18:1) | C23H46NO7P | 479.30 | Ester | Inflammatory signaling |
PC(P-18:0/18:1(9Z)) | C44H86NO7P | 772.15 | Ether | Lipid raft formation |
Table 2: Research Findings in Infection Models
Preparation Methods
General Synthetic Approach
The synthesis of 1-(1Z-octadecenyl)-2-(9Z-octadecenoyl)-sn-glycero-3-phosphoethanolamine typically follows a multi-step process involving:
- Formation of the vinyl ether bond at sn-1 position : This is achieved by starting from 1-(1Z-octadecenyl)-sn-glycerol, which contains the characteristic alk-1-enyl (vinyl ether) group.
- Acylation at the sn-2 position : The introduction of the (9Z)-octadecenoyl (oleoyl) group is performed by acylating the 1-(1Z-octadecenyl)-sn-glycerol intermediate with oleic acid or its activated derivatives (e.g., acid chlorides or anhydrides).
- Attachment of the phosphoethanolamine head group at sn-3 : This step involves phosphorylation and subsequent coupling with ethanolamine to yield the phosphoethanolamine moiety.
This synthetic route ensures the preservation of the sensitive vinyl ether linkage while installing the correct stereochemistry and unsaturation patterns in the fatty acid chains.
Detailed Preparation Steps
Step | Description | Key Reagents/Conditions | Notes |
---|---|---|---|
1 | Preparation of 1-(1Z-octadecenyl)-sn-glycerol | Starting from glycerol derivatives and appropriate alkene precursors under controlled conditions | This step introduces the vinyl ether bond; requires careful control of stereochemistry (cis-configuration) |
2 | Acylation of sn-2 hydroxyl group | Oleic acid or oleoyl chloride, base catalyst (e.g., pyridine), solvent (e.g., dichloromethane), mild temperature control | Ensures selective acylation without degrading vinyl ether; reaction monitored by TLC or HPLC |
3 | Phosphorylation at sn-3 position | Phosphorylating agents such as phosphorus oxychloride (POCl3) or cyclic phosphates, followed by reaction with ethanolamine | Phosphoethanolamine head group introduction; requires neutralization and purification |
4 | Purification | High-performance liquid chromatography (HPLC), silica gel chromatography | Achieves >99% purity; removes unreacted materials and side products |
Reaction Conditions and Optimization
- Temperature and pH control : The acylation and phosphorylation steps are performed under mild temperatures (0–25°C) and controlled pH to prevent hydrolysis or isomerization of the vinyl ether bond.
- Catalysts and solvents : Use of mild bases and aprotic solvents minimizes side reactions.
- Purification : HPLC is essential for obtaining high-purity product suitable for biological assays, eliminating trace impurities that may affect membrane integration studies.
Mechanistic Considerations
- The vinyl ether bond is sensitive to acidic and oxidative conditions; thus, synthesis avoids strong acids and oxidants.
- Acylation is regioselective at the sn-2 hydroxyl due to steric and electronic factors.
- The phosphoethanolamine head group mimics natural phospholipids, facilitating biological relevance.
Representative Data from Research Findings
Parameter | Result/Value | Method/Notes |
---|---|---|
Molecular weight | 730.0 g/mol | Confirmed by mass spectrometry |
Purity | >99% | Determined by HPLC |
Structural confirmation | NMR, IR spectroscopy | Vinyl ether and acyl chain unsaturation confirmed |
Yield | Typically 60-75% overall | Dependent on reaction scale and purification efficiency |
Applications of the Prepared Compound
- Integration into cellular membrane models to study membrane fluidity and permeability.
- Investigation of signaling pathways related to inflammation and cellular stress due to unique vinyl ether linkage.
- Use as a standard or reagent in lipidomics and metabolomics studies involving plasmalogens.
Summary Table of Preparation Methods
Method | Description | Advantages | Challenges |
---|---|---|---|
Acylation of 1-(1Z-octadecenyl)-sn-glycerol with oleic acid derivatives | Direct acylation under mild conditions | High regioselectivity, preserves vinyl ether | Sensitive to reaction conditions; requires careful monitoring |
Phosphorylation and coupling with ethanolamine | Stepwise phosphorylation followed by ethanolamine attachment | Mimics natural head group, biologically relevant | Requires precise control to avoid side reactions |
Purification by HPLC | Chromatographic separation to achieve high purity | Ensures removal of impurities, crucial for biological use | Time-consuming and requires specialized equipment |
Properties
IUPAC Name |
[(2R)-1-[2-aminoethoxy(hydroxy)phosphoryl]oxy-3-[(Z)-octadec-1-enoxy]propan-2-yl] (Z)-octadec-9-enoate | |
---|---|---|
Source | PubChem | |
URL | https://pubchem.ncbi.nlm.nih.gov | |
Description | Data deposited in or computed by PubChem | |
InChI |
InChI=1S/C41H80NO7P/c1-3-5-7-9-11-13-15-17-19-21-23-25-27-29-31-33-36-46-38-40(39-48-50(44,45)47-37-35-42)49-41(43)34-32-30-28-26-24-22-20-18-16-14-12-10-8-6-4-2/h18,20,33,36,40H,3-17,19,21-32,34-35,37-39,42H2,1-2H3,(H,44,45)/b20-18-,36-33-/t40-/m1/s1 | |
Source | PubChem | |
URL | https://pubchem.ncbi.nlm.nih.gov | |
Description | Data deposited in or computed by PubChem | |
InChI Key |
XVYPOHCSLJZFED-QZEVRULJSA-N | |
Source | PubChem | |
URL | https://pubchem.ncbi.nlm.nih.gov | |
Description | Data deposited in or computed by PubChem | |
Canonical SMILES |
CCCCCCCCCCCCCCCCC=COCC(COP(=O)(O)OCCN)OC(=O)CCCCCCCC=CCCCCCCCC | |
Source | PubChem | |
URL | https://pubchem.ncbi.nlm.nih.gov | |
Description | Data deposited in or computed by PubChem | |
Isomeric SMILES |
CCCCCCCCCCCCCCCC/C=C\OC[C@H](COP(=O)(O)OCCN)OC(=O)CCCCCCC/C=C\CCCCCCCC | |
Source | PubChem | |
URL | https://pubchem.ncbi.nlm.nih.gov | |
Description | Data deposited in or computed by PubChem | |
Molecular Formula |
C41H80NO7P | |
Source | PubChem | |
URL | https://pubchem.ncbi.nlm.nih.gov | |
Description | Data deposited in or computed by PubChem | |
DSSTOX Substance ID |
DTXSID301336104 | |
Record name | 1-(1Z-Octadecenyl)-2-(9Z-octadecenoyl)-sn-glycero-3-phosphoethanolamine | |
Source | EPA DSSTox | |
URL | https://comptox.epa.gov/dashboard/DTXSID301336104 | |
Description | DSSTox provides a high quality public chemistry resource for supporting improved predictive toxicology. | |
Molecular Weight |
730.0 g/mol | |
Source | PubChem | |
URL | https://pubchem.ncbi.nlm.nih.gov | |
Description | Data deposited in or computed by PubChem | |
Physical Description |
Solid | |
Record name | PE(P-18:0/18:1(9Z)) | |
Source | Human Metabolome Database (HMDB) | |
URL | http://www.hmdb.ca/metabolites/HMDB0011375 | |
Description | The Human Metabolome Database (HMDB) is a freely available electronic database containing detailed information about small molecule metabolites found in the human body. | |
Explanation | HMDB is offered to the public as a freely available resource. Use and re-distribution of the data, in whole or in part, for commercial purposes requires explicit permission of the authors and explicit acknowledgment of the source material (HMDB) and the original publication (see the HMDB citing page). We ask that users who download significant portions of the database cite the HMDB paper in any resulting publications. | |
CAS No. |
144371-68-6 | |
Record name | 1-(1Z-Octadecenyl)-2-(9Z-octadecenoyl)-sn-glycero-3-phosphoethanolamine | |
Source | EPA DSSTox | |
URL | https://comptox.epa.gov/dashboard/DTXSID301336104 | |
Description | DSSTox provides a high quality public chemistry resource for supporting improved predictive toxicology. | |
Record name | PE(P-18:0/18:1(9Z)) | |
Source | Human Metabolome Database (HMDB) | |
URL | http://www.hmdb.ca/metabolites/HMDB0011375 | |
Description | The Human Metabolome Database (HMDB) is a freely available electronic database containing detailed information about small molecule metabolites found in the human body. | |
Explanation | HMDB is offered to the public as a freely available resource. Use and re-distribution of the data, in whole or in part, for commercial purposes requires explicit permission of the authors and explicit acknowledgment of the source material (HMDB) and the original publication (see the HMDB citing page). We ask that users who download significant portions of the database cite the HMDB paper in any resulting publications. | |
Retrosynthesis Analysis
AI-Powered Synthesis Planning: Our tool employs the Template_relevance Pistachio, Template_relevance Bkms_metabolic, Template_relevance Pistachio_ringbreaker, Template_relevance Reaxys, Template_relevance Reaxys_biocatalysis model, leveraging a vast database of chemical reactions to predict feasible synthetic routes.
One-Step Synthesis Focus: Specifically designed for one-step synthesis, it provides concise and direct routes for your target compounds, streamlining the synthesis process.
Accurate Predictions: Utilizing the extensive PISTACHIO, BKMS_METABOLIC, PISTACHIO_RINGBREAKER, REAXYS, REAXYS_BIOCATALYSIS database, our tool offers high-accuracy predictions, reflecting the latest in chemical research and data.
Strategy Settings
Precursor scoring | Relevance Heuristic |
---|---|
Min. plausibility | 0.01 |
Model | Template_relevance |
Template Set | Pistachio/Bkms_metabolic/Pistachio_ringbreaker/Reaxys/Reaxys_biocatalysis |
Top-N result to add to graph | 6 |
Feasible Synthetic Routes
Disclaimer and Information on In-Vitro Research Products
Please be aware that all articles and product information presented on BenchChem are intended solely for informational purposes. The products available for purchase on BenchChem are specifically designed for in-vitro studies, which are conducted outside of living organisms. In-vitro studies, derived from the Latin term "in glass," involve experiments performed in controlled laboratory settings using cells or tissues. It is important to note that these products are not categorized as medicines or drugs, and they have not received approval from the FDA for the prevention, treatment, or cure of any medical condition, ailment, or disease. We must emphasize that any form of bodily introduction of these products into humans or animals is strictly prohibited by law. It is essential to adhere to these guidelines to ensure compliance with legal and ethical standards in research and experimentation.