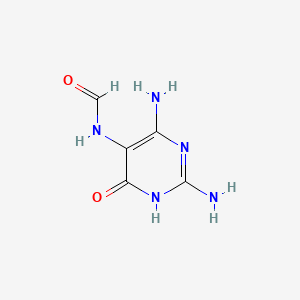
2,4-Diamino-5-(formylamino)-6-hydroxypyrimidine
Overview
Description
2,4-Diamino-5-(formylamino)-6-hydroxypyrimidine is a chemical compound that has been studied for its potential anticancer properties . It is a derivative of 2,4-diamino-5-methyleneaminopyrimidine, which has been shown to have antiproliferative activity .
Synthesis Analysis
The synthesis of 2,4-diamino-5-(formylamino)-6-hydroxypyrimidine derivatives involves a ring-opening strategy . In one study, 25 novel 2,4-diamino-5-methyleneaminopyrimidine derivatives were designed and synthesized .Molecular Structure Analysis
The molecular structure of 2,4-diamino-5-(formylamino)-6-hydroxypyrimidine is based on the 2,4-diamino-5-methyleneaminopyrimidine scaffold . This scaffold has been found to have potential for further investigation to explore novel anticancer agents .Chemical Reactions Analysis
The chemical reactions involved in the synthesis of 2,4-diamino-5-(formylamino)-6-hydroxypyrimidine derivatives are complex and involve multiple steps . These reactions include Knoevenagel condensation, Pinner reactions, and cyclization .Scientific Research Applications
Subheading Inhibition of Microbial Growth
2,4-Diamino-5-(formylamino)-6-hydroxypyrimidine and its derivatives demonstrate inhibitory effects on the growth of various microorganisms, including Streptococcus faecalis, Lactobacillus arabinosus, and Escherichia coli. These compounds interfere with the pyrimidine metabolism of the microorganisms, showcasing their potential as antimicrobial agents (Roy, Ghosh, & Guha, 1961).
DNA Damage and Repair
Subheading Role in DNA Damage and Repair Mechanisms
Formamidopyrimidines like 2,4-Diamino-5-(formylamino)-6-hydroxypyrimidine are formed in DNA by various damaging agents and can be repaired by specific DNA glycosylases. The measurement of these compounds in DNA is crucial for understanding their biological significance and the mechanisms of DNA repair (Jaruga, Kırkalı, & Dizdaroglu, 2008).
Corrosion Inhibition
Subheading Corrosion Inhibition in Industrial Applications
2,4-Diamino-5-(formylamino)-6-hydroxypyrimidine has been evaluated as a corrosion inhibitor for mild steel in acidic mediums. The compound's effectiveness in preventing corrosion is attributed to its adsorption on the metal surface, making it a candidate for applications in corrosion protection (Yıldız, 2018).
Neurochemical Research
Subheading Impact on Neurotransmitter Synthesis
The compound's interference with the synthesis of reduced biopterins affects the function of cerebral tryptophan-5-hydroxylase in vivo. Despite reducing the biopterin pool in the brain, it does not affect the hydroxylation of L-tryptophan or the level of 5-hydroxytryptamine, highlighting the complexity of its role in neurotransmitter synthesis (Em & Whitacre, 1981).
Inhibition of Enzymatic Processes
Subheading Inhibition of GTP Cyclohydrolase I
2,4-Diamino-5-(formylamino)-6-hydroxypyrimidine is known to inhibit GTP cyclohydrolase I, a crucial enzyme in the synthesis of tetrahydrobiopterin. The compound's dual mechanism of inhibition involves direct competition with the substrate and emulation of tetrahydrobiopterin's feedback inhibition system, providing insights into its regulatory effects on enzymatic processes (Xie, Smith, & Gross, 1998).
Spectroscopic Analysis
Subheading Insights from Spectroscopic Studies
Spectrophotometric and vibrational analyses of 2,4-Diamino-5-(formylamino)-6-hydroxypyrimidine provide insights into its molecular structure and dynamics. Studies involving FT-IR and FT-Raman spectra, alongside DFT simulations, offer a comprehensive understanding of the compound's properties and potential applications in various fields (Subramanian, Anbarasan, & Manimegalai, 2009).
Mechanism of Action
Target of Action
The primary target of 2,4-Diamino-5-(formylamino)-6-hydroxypyrimidine is the DNA molecule . It is an oxidized DNA base . The compound interacts with the DNA molecule, specifically with the four canonical nucleic acid bases .
Mode of Action
2,4-Diamino-5-(formylamino)-6-hydroxypyrimidine interacts with its targets by forming pairs with all four canonical nucleic acid bases . In the presence of an external field, the mispairing abilities of 2,4-diamino-5-(formylamino)-6-hydroxypyrimidine become insignificant since the most stable dimers are formed with thymine .
Biochemical Pathways
The biochemical pathways affected by 2,4-Diamino-5-(formylamino)-6-hydroxypyrimidine involve the process of DNA damage. The compound increases the nuclear DNA damage by oxygen-derived radicals .
Result of Action
The molecular and cellular effects of the action of 2,4-Diamino-5-(formylamino)-6-hydroxypyrimidine involve an increase in DNA damage. This is particularly evident in conditions such as Alzheimer’s disease, where the brain is under increased oxidative stress .
Action Environment
The action, efficacy, and stability of 2,4-Diamino-5-(formylamino)-6-hydroxypyrimidine can be influenced by various environmental factors. For instance, the presence of an external field can affect the compound’s ability to form pairs with the four canonical nucleic acid bases
Future Directions
The future directions for research on 2,4-diamino-5-(formylamino)-6-hydroxypyrimidine derivatives are promising. The 2,4-diamino-5-methyleneaminopyrimidine scaffold has potential for further investigation to explore novel anticancer agents . Additionally, these compounds could be studied for their potential as antibacterial agents .
properties
IUPAC Name |
N-(2,4-diamino-6-oxo-1H-pyrimidin-5-yl)formamide | |
---|---|---|
Source | PubChem | |
URL | https://pubchem.ncbi.nlm.nih.gov | |
Description | Data deposited in or computed by PubChem | |
InChI |
InChI=1S/C5H7N5O2/c6-3-2(8-1-11)4(12)10-5(7)9-3/h1H,(H,8,11)(H5,6,7,9,10,12) | |
Source | PubChem | |
URL | https://pubchem.ncbi.nlm.nih.gov | |
Description | Data deposited in or computed by PubChem | |
InChI Key |
RUHWEHKVKUSJAF-UHFFFAOYSA-N | |
Source | PubChem | |
URL | https://pubchem.ncbi.nlm.nih.gov | |
Description | Data deposited in or computed by PubChem | |
Canonical SMILES |
C(=O)NC1=C(N=C(NC1=O)N)N | |
Source | PubChem | |
URL | https://pubchem.ncbi.nlm.nih.gov | |
Description | Data deposited in or computed by PubChem | |
Molecular Formula |
C5H7N5O2 | |
Source | PubChem | |
URL | https://pubchem.ncbi.nlm.nih.gov | |
Description | Data deposited in or computed by PubChem | |
DSSTOX Substance ID |
DTXSID10199090 | |
Record name | Formamide, N-(2,4-diamino-1,6-dihydro-6-oxo-5-pyrimidinyl)- | |
Source | EPA DSSTox | |
URL | https://comptox.epa.gov/dashboard/DTXSID10199090 | |
Description | DSSTox provides a high quality public chemistry resource for supporting improved predictive toxicology. | |
Molecular Weight |
169.14 g/mol | |
Source | PubChem | |
URL | https://pubchem.ncbi.nlm.nih.gov | |
Description | Data deposited in or computed by PubChem | |
Product Name |
2,4-Diamino-5-(formylamino)-6-hydroxypyrimidine | |
CAS RN |
51093-31-3 | |
Record name | Formamide, N-(2,4-diamino-1,6-dihydro-6-oxo-5-pyrimidinyl)- | |
Source | ChemIDplus | |
URL | https://pubchem.ncbi.nlm.nih.gov/substance/?source=chemidplus&sourceid=0051093313 | |
Description | ChemIDplus is a free, web search system that provides access to the structure and nomenclature authority files used for the identification of chemical substances cited in National Library of Medicine (NLM) databases, including the TOXNET system. | |
Record name | Formamide, N-(2,4-diamino-1,6-dihydro-6-oxo-5-pyrimidinyl)- | |
Source | EPA DSSTox | |
URL | https://comptox.epa.gov/dashboard/DTXSID10199090 | |
Description | DSSTox provides a high quality public chemistry resource for supporting improved predictive toxicology. | |
Retrosynthesis Analysis
AI-Powered Synthesis Planning: Our tool employs the Template_relevance Pistachio, Template_relevance Bkms_metabolic, Template_relevance Pistachio_ringbreaker, Template_relevance Reaxys, Template_relevance Reaxys_biocatalysis model, leveraging a vast database of chemical reactions to predict feasible synthetic routes.
One-Step Synthesis Focus: Specifically designed for one-step synthesis, it provides concise and direct routes for your target compounds, streamlining the synthesis process.
Accurate Predictions: Utilizing the extensive PISTACHIO, BKMS_METABOLIC, PISTACHIO_RINGBREAKER, REAXYS, REAXYS_BIOCATALYSIS database, our tool offers high-accuracy predictions, reflecting the latest in chemical research and data.
Strategy Settings
Precursor scoring | Relevance Heuristic |
---|---|
Min. plausibility | 0.01 |
Model | Template_relevance |
Template Set | Pistachio/Bkms_metabolic/Pistachio_ringbreaker/Reaxys/Reaxys_biocatalysis |
Top-N result to add to graph | 6 |
Feasible Synthetic Routes
Disclaimer and Information on In-Vitro Research Products
Please be aware that all articles and product information presented on BenchChem are intended solely for informational purposes. The products available for purchase on BenchChem are specifically designed for in-vitro studies, which are conducted outside of living organisms. In-vitro studies, derived from the Latin term "in glass," involve experiments performed in controlled laboratory settings using cells or tissues. It is important to note that these products are not categorized as medicines or drugs, and they have not received approval from the FDA for the prevention, treatment, or cure of any medical condition, ailment, or disease. We must emphasize that any form of bodily introduction of these products into humans or animals is strictly prohibited by law. It is essential to adhere to these guidelines to ensure compliance with legal and ethical standards in research and experimentation.