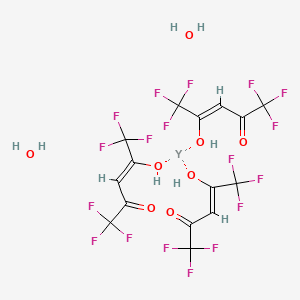
Yttrium hexafluoroacetylacetonate dihydrate
Overview
Description
Yttrium hexafluoroacetylacetonate dihydrate is a coordination complex with the chemical formula Y(C5HF6O2)3·2H2O. This compound consists of yttrium ions coordinated to hexafluoroacetylacetonate ligands and two molecules of water of crystallization. It is a white powder or crystalline solid with a melting point of 128-130°C.
Synthetic Routes and Reaction Conditions:
Direct Reaction: Yttrium chloride hexahydrate reacts with hexafluoroacetylacetone in an aqueous solution to form this compound.
Hydrothermal Synthesis: This method involves reacting yttrium chloride hexahydrate with hexafluoroacetylacetone in a sealed autoclave at elevated temperatures and pressures.
Industrial Production Methods: The industrial production of this compound typically involves large-scale hydrothermal synthesis due to its efficiency and scalability. The process requires precise control of temperature, pressure, and reaction time to ensure high purity and yield.
Types of Reactions:
Oxidation: this compound can undergo oxidation reactions to form yttrium oxides.
Reduction: Reduction reactions can convert this compound to lower oxidation state yttrium compounds.
Substitution: The compound can participate in substitution reactions where the hexafluoroacetylacetonate ligands are replaced by other ligands.
Common Reagents and Conditions:
Oxidation: Oxidizing agents such as hydrogen peroxide or ozone, under controlled conditions.
Reduction: Reducing agents like sodium borohydride or hydrogen gas, typically in an inert atmosphere.
Substitution: Various ligands can be used, depending on the desired product, often in the presence of a solvent.
Major Products Formed:
Oxidation: Yttrium oxide (Y2O3)
Reduction: Yttrium metal or lower oxidation state yttrium compounds
Substitution: Various yttrium complexes with different ligands
Scientific Research Applications
Yttrium hexafluoroacetylacetonate dihydrate is widely used in scientific research due to its unique properties:
Catalysis: It serves as a catalyst in organic synthesis and polymerization reactions.
Material Science: The compound is used in the preparation of yttrium-doped materials for electronic and optical applications.
Biomedical Research: It is employed in the development of yttrium-based imaging agents and therapeutic agents.
Nanotechnology: The compound is utilized in the synthesis of nanomaterials for various applications, including energy storage and conversion.
Mechanism of Action
The mechanism by which yttrium hexafluoroacetylacetonate dihydrate exerts its effects depends on its specific application:
Catalysis: The compound acts as a Lewis acid, facilitating the activation of substrates in chemical reactions.
Material Science: It influences the electronic and optical properties of materials by introducing yttrium ions into the crystal lattice.
Biomedical Research: The yttrium ions can interact with biological targets, leading to imaging or therapeutic effects.
Nanotechnology: The compound helps in the controlled growth of nanomaterials by providing a stable yttrium source.
Comparison with Similar Compounds
Lanthanum Hexafluoroacetylacetonate Dihydrate: Similar coordination chemistry but with lanthanum instead of yttrium.
Cerium Hexafluoroacetylacetonate Dihydrate: Similar to yttrium but with cerium as the central metal ion.
Europium Hexafluoroacetylacetonate Dihydrate: Another lanthanide complex with europium as the central metal ion.
Uniqueness: Yttrium hexafluoroacetylacetonate dihydrate is unique due to its specific yttrium ion coordination, which imparts distinct catalytic, material, and biomedical properties compared to other similar compounds.
Properties
IUPAC Name |
(Z)-1,1,1,5,5,5-hexafluoro-4-hydroxypent-3-en-2-one;yttrium;dihydrate | |
---|---|---|
Source | PubChem | |
URL | https://pubchem.ncbi.nlm.nih.gov | |
Description | Data deposited in or computed by PubChem | |
InChI |
InChI=1S/3C5H2F6O2.2H2O.Y/c3*6-4(7,8)2(12)1-3(13)5(9,10)11;;;/h3*1,12H;2*1H2;/b3*2-1-;;; | |
Source | PubChem | |
URL | https://pubchem.ncbi.nlm.nih.gov | |
Description | Data deposited in or computed by PubChem | |
InChI Key |
FBGQQUQRBZKLQE-OHYMPEQPSA-N | |
Source | PubChem | |
URL | https://pubchem.ncbi.nlm.nih.gov | |
Description | Data deposited in or computed by PubChem | |
Canonical SMILES |
C(=C(C(F)(F)F)O)C(=O)C(F)(F)F.C(=C(C(F)(F)F)O)C(=O)C(F)(F)F.C(=C(C(F)(F)F)O)C(=O)C(F)(F)F.O.O.[Y] | |
Source | PubChem | |
URL | https://pubchem.ncbi.nlm.nih.gov | |
Description | Data deposited in or computed by PubChem | |
Isomeric SMILES |
C(=C(\O)/C(F)(F)F)\C(=O)C(F)(F)F.C(=C(\O)/C(F)(F)F)\C(=O)C(F)(F)F.C(=C(\O)/C(F)(F)F)\C(=O)C(F)(F)F.O.O.[Y] | |
Source | PubChem | |
URL | https://pubchem.ncbi.nlm.nih.gov | |
Description | Data deposited in or computed by PubChem | |
Molecular Formula |
C15H10F18O8Y | |
Source | PubChem | |
URL | https://pubchem.ncbi.nlm.nih.gov | |
Description | Data deposited in or computed by PubChem | |
Molecular Weight |
749.11 g/mol | |
Source | PubChem | |
URL | https://pubchem.ncbi.nlm.nih.gov | |
Description | Data deposited in or computed by PubChem | |
Retrosynthesis Analysis
AI-Powered Synthesis Planning: Our tool employs the Template_relevance Pistachio, Template_relevance Bkms_metabolic, Template_relevance Pistachio_ringbreaker, Template_relevance Reaxys, Template_relevance Reaxys_biocatalysis model, leveraging a vast database of chemical reactions to predict feasible synthetic routes.
One-Step Synthesis Focus: Specifically designed for one-step synthesis, it provides concise and direct routes for your target compounds, streamlining the synthesis process.
Accurate Predictions: Utilizing the extensive PISTACHIO, BKMS_METABOLIC, PISTACHIO_RINGBREAKER, REAXYS, REAXYS_BIOCATALYSIS database, our tool offers high-accuracy predictions, reflecting the latest in chemical research and data.
Strategy Settings
Precursor scoring | Relevance Heuristic |
---|---|
Min. plausibility | 0.01 |
Model | Template_relevance |
Template Set | Pistachio/Bkms_metabolic/Pistachio_ringbreaker/Reaxys/Reaxys_biocatalysis |
Top-N result to add to graph | 6 |
Feasible Synthetic Routes
Q1: What is the role of Yttrium hexafluoroacetylacetonate dihydrate in the fabrication of solid oxide fuel cells?
A1: this compound (Y6FA) serves as a precursor material in the Plasma-enhanced metalorganic chemical vapor deposition (PE-MOCVD) process for fabricating yttria-stabilized zirconia (YSZ) thin films []. YSZ is a crucial component in solid oxide fuel cells (SOFCs), acting as the electrolyte.
Disclaimer and Information on In-Vitro Research Products
Please be aware that all articles and product information presented on BenchChem are intended solely for informational purposes. The products available for purchase on BenchChem are specifically designed for in-vitro studies, which are conducted outside of living organisms. In-vitro studies, derived from the Latin term "in glass," involve experiments performed in controlled laboratory settings using cells or tissues. It is important to note that these products are not categorized as medicines or drugs, and they have not received approval from the FDA for the prevention, treatment, or cure of any medical condition, ailment, or disease. We must emphasize that any form of bodily introduction of these products into humans or animals is strictly prohibited by law. It is essential to adhere to these guidelines to ensure compliance with legal and ethical standards in research and experimentation.