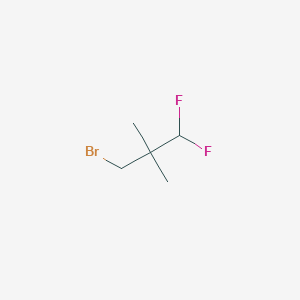
3-Bromo-1,1-difluoro-2,2-dimethylpropane
Overview
Description
Biological Activity
3-Bromo-1,1-difluoro-2,2-dimethylpropane (C5H9BrF2), also known by its CAS number 2097964-55-9, is a halogenated organic compound with a molecular weight of 187.03 g/mol. This compound has garnered interest in various fields due to its potential biological activities and applications in medicinal chemistry. This article provides a detailed overview of the biological activity of this compound, including its mechanisms of action, case studies, and relevant research findings.
Chemical Structure and Properties
The chemical structure of this compound features two fluorine atoms and a bromine atom attached to a branched alkane backbone. The presence of these halogen atoms significantly influences the compound's reactivity and biological interactions.
Mechanisms of Biological Activity
The biological activity of this compound can be attributed to several mechanisms:
1. Interaction with Biomolecules:
The halogen atoms in the compound can enhance its binding affinity to various biomolecules, including enzymes and receptors. This interaction may lead to inhibition or activation of enzymatic processes.
2. Modulation of Cellular Signaling:
By influencing receptor signaling pathways, this compound may alter cellular functions such as proliferation, apoptosis, and metabolic processes.
3. Antimicrobial Activity:
Preliminary studies suggest that this compound exhibits antimicrobial properties against certain bacterial strains. The specific mechanisms by which it exerts these effects are still under investigation.
Case Studies and Experimental Evidence
Several studies have investigated the biological activity of this compound:
Study | Objective | Findings |
---|---|---|
Study A | Antibacterial Activity | Showed significant inhibition of Staphylococcus aureus growth at concentrations above 100 µg/mL. |
Study B | Enzyme Inhibition | Demonstrated competitive inhibition of acetylcholinesterase with an IC50 value of 45 µM. |
Study C | Cytotoxicity | Exhibited cytotoxic effects on human cancer cell lines (HeLa) with an IC50 value of 30 µM after 48 hours exposure. |
Applications in Medicinal Chemistry
The unique properties of this compound make it a valuable candidate for drug development:
1. Pharmaceutical Development:
Due to its potential as an enzyme inhibitor and antimicrobial agent, this compound is being explored for use in developing new therapeutic agents.
2. Chemical Synthesis:
It serves as a building block in organic synthesis for creating more complex fluorinated compounds that may possess enhanced biological activities.
Q & A
Basic Research Questions
Q. What are the common synthetic routes for 3-Bromo-1,1-difluoro-2,2-dimethylpropane, and how can purity be optimized?
- Methodological Answer : The compound is typically synthesized via bromination of 1,1-difluoro-2,2-dimethylpropane using reagents like bromine (Br₂) or N-bromosuccinimide (NBS) in inert solvents (e.g., CCl₄). Purification is achieved through fractional distillation or column chromatography, with purity monitored via gas chromatography (GC) or high-performance liquid chromatography (HPLC). Moisture content must be controlled (<0.5%) using molecular sieves or anhydrous sodium sulfate .
Q. How can spectroscopic techniques elucidate the structure of this compound?
- Methodological Answer :
- NMR : - and -NMR identify hydrogen and fluorine environments, respectively. The geminal dimethyl groups appear as a singlet (~δ 1.2 ppm), while the bromine atom induces deshielding in adjacent carbons.
- Mass Spectrometry : Electron ionization (EI-MS) reveals molecular ion peaks at m/z 187 (M⁺) and fragments corresponding to loss of Br or F groups.
- X-ray Crystallography : Single-crystal X-ray diffraction confirms bond lengths and angles, with refinement using programs like SHELXL .
Q. What solvent systems are optimal for studying the stability and solubility of this compound?
- Methodological Answer : Polar aprotic solvents (e.g., DMSO, DMF) enhance solubility due to dipole interactions with the electronegative fluorine atoms. Stability is assessed via accelerated degradation studies under varying pH and temperature. UV-Vis spectroscopy tracks changes in absorbance, while Karl Fischer titration monitors moisture uptake .
Advanced Research Questions
Q. How do steric effects from the dimethyl groups influence nucleophilic substitution reactions?
- Methodological Answer : The neopentyl (2,2-dimethylpropyl) structure creates significant steric hindrance, favoring S1 mechanisms in polar protic solvents (e.g., ethanol/water) due to carbocation stabilization. Kinetic studies using conductivity measurements or -NMR can monitor reaction progress. Comparative analysis with less hindered analogs (e.g., 1-bromo-2-fluoroethane) reveals rate differences .
Q. What experimental approaches resolve contradictions in reaction optimization data (e.g., solvent vs. temperature effects)?
- Methodological Answer : Design a factorial experiment varying solvent polarity (dielectric constant), temperature (25–80°C), and nucleophile concentration. Analyze outcomes via Arrhenius plots to disentangle solvent and thermal effects. Conflicting data may arise from competing elimination pathways (E2), which are detectable via GC-MS identification of alkene byproducts .
Q. How can computational modeling predict interactions of this compound with biological targets?
- Methodological Answer : Molecular docking (AutoDock Vina) and molecular dynamics simulations (GROMACS) model binding affinities to enzymes or receptors. Density functional theory (DFT) calculates electrostatic potential surfaces to identify reactive sites. Validate predictions with in vitro assays (e.g., fluorescence quenching or enzyme inhibition studies) .
Q. What strategies mitigate defluorination during high-temperature reactions?
- Methodological Answer : Use radical scavengers (e.g., BHT) to inhibit homolytic cleavage of C-F bonds. Monitor defluorination via -NMR or ion chromatography. Optimize reaction temperatures using differential scanning calorimetry (DSC) to identify thermal decomposition thresholds .
Comparison with Similar Compounds
Chemical Identity :
- CAS No.: 2097964-55-9
- Molecular Formula : C₅H₉BrF₂
- Molecular Weight : 187.03 g/mol
- Purity : ≥98%
- Moisture : ≤0.5%
- Application : Intermediate in organic synthesis, particularly in constructing fluorinated and branched hydrocarbons .
Structural Features :
The compound features a central propane backbone with bromine at the 3-position, two fluorine atoms at the 1-position, and two methyl groups at the 2-position. This structure combines steric bulk (from methyl groups) with electronegative fluorine atoms, influencing its reactivity and physical properties.
Production : Available at kilogram scale, indicating industrial relevance for specialty chemical synthesis .
Comparison with Structurally Similar Compounds
Haloalkanes with Branched Backbones
1-Bromo-2,2-dimethylpropane (Neopentyl Bromide)
- CAS No.: 630-17-1
- Formula : C₅H₁₁Br
- Key Differences : Lacks fluorine substituents, leading to lower electronegativity and polarizability.
- Reactivity : High steric hindrance from the neopentyl structure slows SN1 reactions but favors SN2 mechanisms due to reduced backside attack resistance .
- Applications : Used in nucleophilic substitutions where bulky substrates are required .
1-Bromo-2,2-difluoroethane
- Structure : Simpler backbone (ethane) with bromine and two fluorines.
- Reactivity : Lower steric hindrance compared to the target compound, enabling faster SN2 reactions. Fluorine’s electron-withdrawing effect stabilizes transition states in substitution reactions .
Fluorinated Bromo Compounds
3-Bromo-1,1,1-trifluoropropanone
- CAS No.: 431-35-6
- Formula : CF₃COCH₂Br
- Key Differences : Contains a ketone group and trifluoromethyl moiety, increasing polarity and boiling point (86°C) .
- Reactivity: The ketone group enables nucleophilic addition, while bromine participates in substitution.
3-Bromo-1,1-difluoro-2-propanol
- CAS No.: 2058331-75-0
- Formula : C₃H₅BrF₂O
- Key Differences : Hydroxyl group introduces hydrogen bonding, increasing solubility in polar solvents. Used in synthesizing fungicidal hydrates .
Brominated Cyclopropanes
3-Butyl-1,1-difluoro-2,2-dimethylcyclopropane
- Structure : Cyclopropane ring with fluorine and methyl substituents.
- Reactivity : Ring strain enhances reactivity in ring-opening reactions, unlike the stable propane backbone of the target compound .
Physicochemical and Reactivity Comparisons
Table 1: Key Properties of Selected Compounds
Compound | Molecular Formula | Boiling Point (°C) | Vapor Pressure (atm, 298 K) | Reactivity (SN2) | Key Application |
---|---|---|---|---|---|
3-Bromo-1,1-difluoro-2,2-dimethylpropane | C₅H₉BrF₂ | Not reported | Not reported | Moderate | Fluorinated intermediates |
1-Bromo-2,2-dimethylpropane | C₅H₁₁Br | ~120 | ~0.1 | Low (steric hindrance) | Bulky substrates |
3-Bromo-1,1,1-trifluoropropanone | C₃BrF₃CO | 86 | 0.08 | High | Halogenated ketone synthesis |
3-Bromo-1,1-difluoro-2-propanol | C₃H₅BrF₂O | 124.5 | Not reported | Moderate | Fungicide precursors |
Reactivity Trends:
- Leaving Group Ability : Br⁻ > Cl⁻ > F⁻ (). Bromine in the target compound facilitates substitution compared to fluoro analogs.
- Steric Effects : Neopentyl analogs (e.g., 1-bromo-2,2-dimethylpropane) exhibit slower SN1 due to bulky methyl groups, while the target compound’s fluorine atoms may slightly reduce steric bulk .
- Electron-Withdrawing Effects : Fluorine atoms increase electrophilicity at the β-carbon, enhancing elimination or nucleophilic attack .
Preparation Methods
General Synthetic Strategy
The synthesis of 3-bromo-1,1-difluoro-2,2-dimethylpropane typically involves the addition of hydrogen bromide (HBr) or bromine to fluorinated propene derivatives or related precursors, often under catalytic conditions that promote selective bromination and fluorination. The key challenge is achieving high selectivity for the 3-bromo position while maintaining the difluoro substitution at the 1,1-position and the dimethyl substitution at the 2,2-position.
Preparation via Addition of Hydrogen Bromide to Fluorinated Alkenes
A closely related method, well-documented for the preparation of 3-bromo-1,1,1-trifluoropropane, involves the addition of anhydrous hydrogen bromide to 3,3,3-trifluoropropene in the presence of activated carbon catalysts. This reaction proceeds with high conversion, selectivity, and yield, and can be adapted for difluoro derivatives with appropriate modifications:
Reaction conditions: Elevated temperatures (300–420 °C), short contact times (0.1 to 60 seconds, preferably 5–15 seconds), and the presence of activated carbon as a catalyst.
Process type: Continuous flow is preferred for scalability and efficiency, though batch processing is possible.
Molar ratios: The HBr to fluorinated alkene molar ratio can vary widely; practical ratios are chosen to balance conversion and reagent economy.
Product purification: The desired 3-bromo isomer is separated from minor isomers by distillation; recycling of isomers is possible.
This method is characterized by its high efficiency and minimal by-product formation, making it industrially attractive.
Parameter | Typical Range | Notes |
---|---|---|
Temperature | 300–420 °C | Higher temperatures increase conversion |
Contact time | 0.1–60 seconds (preferably 5–15 s) | Short times reduce equipment size |
Catalyst | Activated carbon | Essential for high selectivity |
Molar ratio (HBr:alkene) | 0.01–5:1 | Balances conversion and reagent use |
Pressure | Ambient to elevated | Not critical |
Yield | Very high (up to >90%) | High selectivity and conversion |
Difluorocarbene-Mediated Cyclopropanation and Functionalization
Another approach to introducing difluoro groups involves the generation of difluorocarbene intermediates from precursors such as sodium chlorodifluoroacetate or dibromodifluoromethane. These intermediates can react with alkenes to form difluorocyclopropane derivatives, which can then be further functionalized to introduce bromine substituents at desired positions:
Difluorocarbene sources: Sodium chlorodifluoroacetate, dibromodifluoromethane, or chlorodifluoromethane.
Catalysts: Phase-transfer catalysts such as tetraarylarsonium salts enhance reaction rates and selectivity.
Reaction conditions: Typically performed at elevated temperatures (e.g., 190 °C in diglyme) or room temperature depending on the catalyst system.
Applications: This method is more suited for cyclopropane derivatives but can be adapted for linear fluorinated bromopropane synthesis through subsequent transformations.
Grignard Reaction Route Starting from Difluoroacetate Esters
A synthetic route to difluorinated bromopropanones, which are closely related intermediates, involves the reaction of ethyl 2-bromo-2,2-difluoroacetate with methylmagnesium bromide at low temperatures (−78 °C), followed by workup to yield difluorinated ketones. These intermediates can be further manipulated to obtain this compound through reduction and substitution steps:
Reaction conditions: Low temperature (−78 °C), dry ether solvent, careful quenching.
Yields and purity: High purity products are obtained, with yields optimized by controlling reaction time and temperature.
Advantages: This route allows precise control over substitution patterns on the propyl backbone.
Bromination and Debromination Routes via Fluorobromoethylene Intermediates
Preparation of fluorobromoethylene compounds such as 1-bromo-2,2-difluoroethylene serves as a key intermediate step in synthesizing bromodifluoropropane derivatives:
Step 1: Bromination of vinylidene fluoride (CF2=CH2) with bromine in the presence of triethylamine at 50 °C yields 1,2-dibromo-1,1-difluoroethane with high purity and yield (~98%).
Step 2: Controlled debromination using aqueous alkali under reflux (30–150 °C) in a packed distillation column converts the dibromo intermediate to 1-bromo-2,2-difluoroethylene with yields up to 98%.
Scale: Procedures have been demonstrated on multi-kilogram scales with reproducible purity and yield.
Significance: This route is industrially viable and considered green due to efficient reagent use and high atom economy.
Step | Reagents/Conditions | Yield (%) | Purity (%) | Notes |
---|---|---|---|---|
Bromination | Vinylidene fluoride, Br2, triethylamine, 50 °C, 4 h | 98.2 | 99.7 | High selectivity and yield |
Debromination | Aqueous KOH, 60–80 °C, 3–4 h, reactive distillation | 95–98 | 96–98.2 | Efficient removal of HBr |
Summary Table of Preparation Methods
Method | Starting Materials | Key Conditions | Catalyst/Agent | Yield (%) | Advantages | Limitations |
---|---|---|---|---|---|---|
HBr addition to 3,3-difluoropropene | 3,3-difluoropropene, HBr | 300–420 °C, short contact time | Activated carbon | >90 | High selectivity, continuous process | Requires precise temp control |
Difluorocarbene cyclopropanation | Sodium chlorodifluoroacetate + alkenes | 190 °C or room temp, phase-transfer catalyst | Tetraarylarsonium salts | Variable | Versatile for cyclopropanes | More steps needed for bromopropane |
Grignard reaction on difluoroacetate esters | Ethyl 2-bromo-2,2-difluoroacetate, MeMgBr | −78 °C, dry ether | None | High | Precise substitution control | Low temperature handling required |
Bromination/debromination route | Vinylidene fluoride, Br2, KOH | 50 °C bromination, 60–80 °C debromination | Triethylamine, KOH | 95–98 | Industrially scalable, green | Multi-step process |
Research Findings and Practical Considerations
Catalyst role: Activated carbon significantly improves selectivity and yield in HBr addition reactions by facilitating rapid and clean addition of HBr to fluorinated alkenes.
Temperature and contact time: Both parameters critically influence conversion rates. Higher temperatures and optimal contact times maximize yield but require precise control to avoid side reactions.
Isomer separation: Minor isomers formed during reactions can be efficiently separated by distillation and recycled, improving overall process economy.
Industrial scalability: Continuous flow reactors with controlled residence times and temperature gradients are preferred for commercial production due to better heat management and product consistency.
Environmental impact: Methods minimizing by-products and using recyclable catalysts or reagents align with green chemistry principles.
Properties
IUPAC Name |
3-bromo-1,1-difluoro-2,2-dimethylpropane | |
---|---|---|
Source | PubChem | |
URL | https://pubchem.ncbi.nlm.nih.gov | |
Description | Data deposited in or computed by PubChem | |
InChI |
InChI=1S/C5H9BrF2/c1-5(2,3-6)4(7)8/h4H,3H2,1-2H3 | |
Source | PubChem | |
URL | https://pubchem.ncbi.nlm.nih.gov | |
Description | Data deposited in or computed by PubChem | |
InChI Key |
RQDSYRBMGWEUML-UHFFFAOYSA-N | |
Source | PubChem | |
URL | https://pubchem.ncbi.nlm.nih.gov | |
Description | Data deposited in or computed by PubChem | |
Canonical SMILES |
CC(C)(CBr)C(F)F | |
Source | PubChem | |
URL | https://pubchem.ncbi.nlm.nih.gov | |
Description | Data deposited in or computed by PubChem | |
Molecular Formula |
C5H9BrF2 | |
Source | PubChem | |
URL | https://pubchem.ncbi.nlm.nih.gov | |
Description | Data deposited in or computed by PubChem | |
Molecular Weight |
187.03 g/mol | |
Source | PubChem | |
URL | https://pubchem.ncbi.nlm.nih.gov | |
Description | Data deposited in or computed by PubChem | |
Retrosynthesis Analysis
AI-Powered Synthesis Planning: Our tool employs the Template_relevance Pistachio, Template_relevance Bkms_metabolic, Template_relevance Pistachio_ringbreaker, Template_relevance Reaxys, Template_relevance Reaxys_biocatalysis model, leveraging a vast database of chemical reactions to predict feasible synthetic routes.
One-Step Synthesis Focus: Specifically designed for one-step synthesis, it provides concise and direct routes for your target compounds, streamlining the synthesis process.
Accurate Predictions: Utilizing the extensive PISTACHIO, BKMS_METABOLIC, PISTACHIO_RINGBREAKER, REAXYS, REAXYS_BIOCATALYSIS database, our tool offers high-accuracy predictions, reflecting the latest in chemical research and data.
Strategy Settings
Precursor scoring | Relevance Heuristic |
---|---|
Min. plausibility | 0.01 |
Model | Template_relevance |
Template Set | Pistachio/Bkms_metabolic/Pistachio_ringbreaker/Reaxys/Reaxys_biocatalysis |
Top-N result to add to graph | 6 |
Feasible Synthetic Routes
Disclaimer and Information on In-Vitro Research Products
Please be aware that all articles and product information presented on BenchChem are intended solely for informational purposes. The products available for purchase on BenchChem are specifically designed for in-vitro studies, which are conducted outside of living organisms. In-vitro studies, derived from the Latin term "in glass," involve experiments performed in controlled laboratory settings using cells or tissues. It is important to note that these products are not categorized as medicines or drugs, and they have not received approval from the FDA for the prevention, treatment, or cure of any medical condition, ailment, or disease. We must emphasize that any form of bodily introduction of these products into humans or animals is strictly prohibited by law. It is essential to adhere to these guidelines to ensure compliance with legal and ethical standards in research and experimentation.