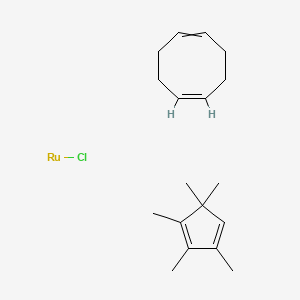
Chloro(pentamethylcyclopentadienyl)(cyclooctadiene)ruthenium(II)
- Click on QUICK INQUIRY to receive a quote from our team of experts.
- With the quality product at a COMPETITIVE price, you can focus more on your research.
Description
Chloro(pentamethylcyclopentadienyl)(cyclooctadiene)ruthenium(II) is a useful research compound. Its molecular formula is C18H28ClRu and its molecular weight is 380.9 g/mol. The purity is usually 95%.
BenchChem offers high-quality Chloro(pentamethylcyclopentadienyl)(cyclooctadiene)ruthenium(II) suitable for many research applications. Different packaging options are available to accommodate customers' requirements. Please inquire for more information about Chloro(pentamethylcyclopentadienyl)(cyclooctadiene)ruthenium(II) including the price, delivery time, and more detailed information at info@benchchem.com.
Mechanism of Action
Target of Action
Chloro(pentamethylcyclopentadienyl)(cyclooctadiene)ruthenium(II) is a homogeneous catalyst that primarily targets the formation of carbon-carbon and carbon-heteroatom bonds . It plays a crucial role in facilitating various chemical reactions, particularly those involving alkynylboronates, propargyl alcohols, and terminal alkynes .
Mode of Action
The compound interacts with its targets by catalyzing the cyclotrimerization of alkynylboronates, propargyl alcohols, and terminal alkynes . This interaction leads to the formation of arylboronate . The arylboronate then undergoes palladium(II)-catalyzed carbonylation .
Biochemical Pathways
The biochemical pathways affected by Chloro(pentamethylcyclopentadienyl)(cyclooctadiene)ruthenium(II) primarily involve the formation of carbon-carbon and carbon-heteroatom bonds . The compound catalyzes the cyclotrimerization of alkynylboronates, propargyl alcohols, and terminal alkynes to form arylboronate . This arylboronate then undergoes palladium(II)-catalyzed carbonylation, leading to the formation of highly substituted phthalides .
Result of Action
The molecular and cellular effects of Chloro(pentamethylcyclopentadienyl)(cyclooctadiene)ruthenium(II)'s action primarily involve the formation of carbon-carbon and carbon-heteroatom bonds . This leads to the creation of new compounds, such as highly substituted phthalides , which can have various applications in chemical synthesis.
Action Environment
The action, efficacy, and stability of Chloro(pentamethylcyclopentadienyl)(cyclooctadiene)ruthenium(II) can be influenced by various environmental factors. For instance, the compound is stable at room temperature but may decompose when heated . It is soluble in organic solvents like dichloromethane and ether, but only slightly soluble in water . These properties can affect the compound’s performance as a catalyst in different reaction environments.
Properties
CAS No. |
92390-26-6 |
---|---|
Molecular Formula |
C18H28ClRu |
Molecular Weight |
380.9 g/mol |
IUPAC Name |
chlororuthenium;(5Z)-cycloocta-1,5-diene;1,2,3,5,5-pentamethylcyclopenta-1,3-diene |
InChI |
InChI=1S/C10H16.C8H12.ClH.Ru/c1-7-6-10(4,5)9(3)8(7)2;1-2-4-6-8-7-5-3-1;;/h6H,1-5H3;1-2,7-8H,3-6H2;1H;/q;;;+1/p-1/b;2-1-,8-7?;; |
InChI Key |
JBVMVFXUVNUNNG-ZNZJSHLGSA-M |
Isomeric SMILES |
CC1=CC(C(=C1C)C)(C)C.C1C/C=C\CCC=C1.Cl[Ru] |
SMILES |
CC1=CC(C(=C1C)C)(C)C.C1CC=CCCC=C1.Cl[Ru] |
Canonical SMILES |
CC1=CC(C(=C1C)C)(C)C.C1CC=CCCC=C1.Cl[Ru] |
Origin of Product |
United States |
Q1: What makes chloro(pentamethylcyclopentadienyl)(cyclooctadiene)ruthenium(II) a suitable catalyst for this specific [2+2+2] cycloaddition reaction?
A1: While the paper doesn't delve into the specific mechanistic advantages of chloro(pentamethylcyclopentadienyl)(cyclooctadiene)ruthenium(II), it highlights its superior performance compared to other transition metal-based catalysts for this reaction []. This suggests that the steric and electronic properties conferred by the pentamethylcyclopentadienyl and cyclooctadiene ligands likely contribute to favorable interactions with the substrates (diynes and N-cyanoindoles), facilitating the formation of the desired 1-(2-pyridyl)indole products. Further research into the reaction mechanism and comparative studies with other catalysts would be needed to provide a more definitive answer.
Disclaimer and Information on In-Vitro Research Products
Please be aware that all articles and product information presented on BenchChem are intended solely for informational purposes. The products available for purchase on BenchChem are specifically designed for in-vitro studies, which are conducted outside of living organisms. In-vitro studies, derived from the Latin term "in glass," involve experiments performed in controlled laboratory settings using cells or tissues. It is important to note that these products are not categorized as medicines or drugs, and they have not received approval from the FDA for the prevention, treatment, or cure of any medical condition, ailment, or disease. We must emphasize that any form of bodily introduction of these products into humans or animals is strictly prohibited by law. It is essential to adhere to these guidelines to ensure compliance with legal and ethical standards in research and experimentation.