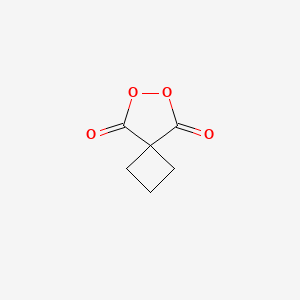
Cyclobutane malonyl peroxide
Overview
Description
Cyclobutane malonyl peroxide is a useful research compound. Its molecular formula is C6H6O4 and its molecular weight is 142.11 g/mol. The purity is usually 95%.
BenchChem offers high-quality this compound suitable for many research applications. Different packaging options are available to accommodate customers' requirements. Please inquire for more information about this compound including the price, delivery time, and more detailed information at info@benchchem.com.
Mechanism of Action
Target of Action
Cyclobutane malonyl peroxide primarily targets alkenes . Alkenes are unsaturated hydrocarbons that contain at least one carbon-carbon double bond. They play a crucial role in organic chemistry as they are often used as building blocks to create more complex molecules .
Mode of Action
This compound interacts with its targets through a process known as dioxygenation . This process involves the addition of oxygen atoms to the alkene, resulting in the formation of diols or heterocyclic compounds . The reaction of alkenes with this compound in the presence of water leads to the formation of syn-1,2-diols . This reaction is followed by hydrolysis, which breaks down the compound into simpler substances .
Biochemical Pathways
The primary biochemical pathway affected by this compound is the oxidative C–O coupling with arenes, enol ethers, 1,3-dicarbonyl, and N-heterocyclic compounds . This pathway involves the sequential decarboxylative condensation of five molecules of malonyl-coenzyme A . The end products of this pathway include diols and heterocyclic compounds .
Result of Action
The molecular and cellular effects of this compound’s action primarily involve the formation of diols or heterocyclic compounds through the dioxygenation of alkenes . These compounds can have various biological activities and can serve as precursors for the synthesis of more complex molecules . In addition, one of the isomers of this compound has shown excellent in vitro anti-cancer activity towards various cancer cell lines .
Action Environment
The action, efficacy, and stability of this compound can be influenced by various environmental factors. For instance, the presence of water is necessary for the dioxygenation of alkenes . Additionally, the reaction medium can also affect the yield and selectivity of the reaction . More research is needed to fully understand how different environmental factors influence the action of this compound.
Biochemical Analysis
Biochemical Properties
Cyclobutane malonyl peroxide plays a significant role in biochemical reactions, particularly in oxidative processes. It interacts with various enzymes, proteins, and other biomolecules. One of the primary interactions is with peroxidase enzymes, which catalyze the decomposition of peroxides. This compound can act as a substrate for these enzymes, leading to the formation of reactive oxygen species (ROS). These ROS can further interact with other biomolecules, leading to oxidative modifications. Additionally, this compound can interact with proteins containing thiol groups, leading to the formation of disulfide bonds and subsequent changes in protein structure and function .
Cellular Effects
This compound has been shown to affect various types of cells and cellular processes. It influences cell function by modulating cell signaling pathways, gene expression, and cellular metabolism. For instance, the presence of this compound can lead to the activation of oxidative stress pathways, resulting in the upregulation of antioxidant genes. This compound can also affect cellular metabolism by altering the activity of key metabolic enzymes, leading to changes in the levels of metabolites such as ATP and NADPH .
Molecular Mechanism
The molecular mechanism of action of this compound involves its interaction with biomolecules at the molecular level. This compound can bind to specific sites on enzymes, leading to either inhibition or activation of their activity. For example, it can inhibit the activity of enzymes involved in fatty acid synthesis by binding to their active sites. Additionally, this compound can induce changes in gene expression by interacting with transcription factors and other regulatory proteins. These interactions can lead to the activation or repression of specific genes, resulting in changes in cellular function .
Temporal Effects in Laboratory Settings
In laboratory settings, the effects of this compound can change over time. The stability of this compound is a critical factor, as it can degrade over time, leading to a decrease in its activity. Studies have shown that this compound can undergo hydrolysis, resulting in the formation of malonic acid and other degradation products. These degradation products can have different effects on cellular function compared to the parent compound. Long-term exposure to this compound in in vitro or in vivo studies has shown that it can lead to chronic oxidative stress, resulting in cellular damage and dysfunction .
Dosage Effects in Animal Models
The effects of this compound vary with different dosages in animal models. At low doses, this compound can induce mild oxidative stress, leading to the activation of protective antioxidant pathways. At high doses, this compound can cause significant oxidative damage, leading to cell death and tissue injury. Threshold effects have been observed, where a specific dosage range results in a switch from protective to harmful effects. Toxic or adverse effects at high doses include liver and kidney damage, as well as impaired immune function .
Metabolic Pathways
This compound is involved in various metabolic pathways, including those related to oxidative stress and fatty acid metabolism. It interacts with enzymes such as acetyl-CoA carboxylase and fatty acid synthase, leading to changes in the levels of key metabolites. This compound can also affect metabolic flux by altering the activity of enzymes involved in the tricarboxylic acid cycle and glycolysis. These changes can result in altered energy production and metabolic homeostasis .
Transport and Distribution
Within cells and tissues, this compound is transported and distributed through various mechanisms. It can interact with transporters and binding proteins that facilitate its movement across cellular membranes. Once inside the cell, this compound can accumulate in specific compartments, such as the mitochondria and endoplasmic reticulum. This localization can influence its activity and function, as different cellular compartments have distinct biochemical environments .
Subcellular Localization
The subcellular localization of this compound plays a crucial role in its activity and function. This compound can be directed to specific compartments or organelles through targeting signals or post-translational modifications. For example, this compound can be targeted to the mitochondria, where it can interact with mitochondrial enzymes and affect cellular respiration. Additionally, its localization to the endoplasmic reticulum can influence protein folding and secretion processes .
Properties
IUPAC Name |
6,7-dioxaspiro[3.4]octane-5,8-dione | |
---|---|---|
Source | PubChem | |
URL | https://pubchem.ncbi.nlm.nih.gov | |
Description | Data deposited in or computed by PubChem | |
InChI |
InChI=1S/C6H6O4/c7-4-6(2-1-3-6)5(8)10-9-4/h1-3H2 | |
Source | PubChem | |
URL | https://pubchem.ncbi.nlm.nih.gov | |
Description | Data deposited in or computed by PubChem | |
InChI Key |
BSQQTMMSCSSKST-UHFFFAOYSA-N | |
Source | PubChem | |
URL | https://pubchem.ncbi.nlm.nih.gov | |
Description | Data deposited in or computed by PubChem | |
Canonical SMILES |
C1CC2(C1)C(=O)OOC2=O | |
Source | PubChem | |
URL | https://pubchem.ncbi.nlm.nih.gov | |
Description | Data deposited in or computed by PubChem | |
Molecular Formula |
C6H6O4 | |
Source | PubChem | |
URL | https://pubchem.ncbi.nlm.nih.gov | |
Description | Data deposited in or computed by PubChem | |
Molecular Weight |
142.11 g/mol | |
Source | PubChem | |
URL | https://pubchem.ncbi.nlm.nih.gov | |
Description | Data deposited in or computed by PubChem | |
Retrosynthesis Analysis
AI-Powered Synthesis Planning: Our tool employs the Template_relevance Pistachio, Template_relevance Bkms_metabolic, Template_relevance Pistachio_ringbreaker, Template_relevance Reaxys, Template_relevance Reaxys_biocatalysis model, leveraging a vast database of chemical reactions to predict feasible synthetic routes.
One-Step Synthesis Focus: Specifically designed for one-step synthesis, it provides concise and direct routes for your target compounds, streamlining the synthesis process.
Accurate Predictions: Utilizing the extensive PISTACHIO, BKMS_METABOLIC, PISTACHIO_RINGBREAKER, REAXYS, REAXYS_BIOCATALYSIS database, our tool offers high-accuracy predictions, reflecting the latest in chemical research and data.
Strategy Settings
Precursor scoring | Relevance Heuristic |
---|---|
Min. plausibility | 0.01 |
Model | Template_relevance |
Template Set | Pistachio/Bkms_metabolic/Pistachio_ringbreaker/Reaxys/Reaxys_biocatalysis |
Top-N result to add to graph | 6 |
Feasible Synthetic Routes
Disclaimer and Information on In-Vitro Research Products
Please be aware that all articles and product information presented on BenchChem are intended solely for informational purposes. The products available for purchase on BenchChem are specifically designed for in-vitro studies, which are conducted outside of living organisms. In-vitro studies, derived from the Latin term "in glass," involve experiments performed in controlled laboratory settings using cells or tissues. It is important to note that these products are not categorized as medicines or drugs, and they have not received approval from the FDA for the prevention, treatment, or cure of any medical condition, ailment, or disease. We must emphasize that any form of bodily introduction of these products into humans or animals is strictly prohibited by law. It is essential to adhere to these guidelines to ensure compliance with legal and ethical standards in research and experimentation.