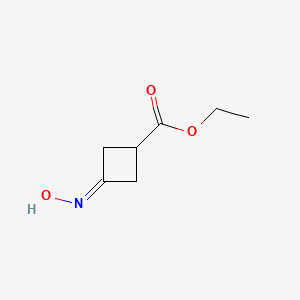
Ethyl 3-(hydroxyimino)cyclobutane-1-carboxylate
Overview
Description
It is a derivative of isoxazole and is commonly used in medical, environmental, and industrial research.
Preparation Methods
Synthetic Routes and Reaction Conditions
The synthesis of Ethyl 3-(hydroxyimino)cyclobutane-1-carboxylate typically involves the reaction of ethyl cyclobutanecarboxylate with hydroxylamine . The reaction conditions often include the use of a solvent such as ethanol and a catalyst to facilitate the reaction. The process may also involve heating the reaction mixture to a specific temperature to ensure complete conversion of the reactants to the desired product .
Industrial Production Methods
In industrial settings, the production of this compound may involve large-scale batch reactors where the reactants are mixed and heated under controlled conditions. The use of automated systems ensures precise control over reaction parameters, leading to high yields and purity of the final product .
Chemical Reactions Analysis
Types of Reactions
Ethyl 3-(hydroxyimino)cyclobutane-1-carboxylate undergoes various chemical reactions, including:
Oxidation: The compound can be oxidized to form corresponding oximes or nitriles.
Reduction: Reduction reactions can convert the compound into amines or other reduced forms.
Substitution: The compound can undergo nucleophilic substitution reactions, where the hydroxyimino group is replaced by other functional groups.
Common Reagents and Conditions
Oxidation: Common oxidizing agents include potassium permanganate and hydrogen peroxide.
Reduction: Reducing agents such as lithium aluminum hydride and sodium borohydride are often used.
Substitution: Nucleophiles like amines, thiols, and alcohols can be used under basic or acidic conditions.
Major Products Formed
Oxidation: Formation of oximes or nitriles.
Reduction: Formation of amines.
Substitution: Formation of substituted cyclobutanecarboxylates.
Scientific Research Applications
Ethyl 3-(hydroxyimino)cyclobutane-1-carboxylate has a wide range of applications in scientific research:
Chemistry: Used as a building block in the synthesis of complex organic molecules.
Biology: Investigated for its potential as a biochemical probe.
Medicine: Explored for its potential therapeutic properties, including anti-inflammatory and antimicrobial activities.
Industry: Utilized in the production of specialty chemicals and materials.
Mechanism of Action
The mechanism of action of Ethyl 3-(hydroxyimino)cyclobutane-1-carboxylate involves its interaction with specific molecular targets and pathways. The compound can act as an inhibitor or activator of certain enzymes, leading to changes in biochemical pathways. The hydroxyimino group plays a crucial role in its reactivity and interaction with biological molecules.
Comparison with Similar Compounds
Ethyl 3-(hydroxyimino)cyclobutane-1-carboxylate can be compared with other similar compounds, such as:
Ethyl cyclobutanecarboxylate: Lacks the hydroxyimino group, leading to different reactivity and applications.
Cyclobutanecarbonyl chloride: Contains a carbonyl chloride group instead of the hydroxyimino group, resulting in different chemical properties and uses.
Properties
IUPAC Name |
ethyl 3-hydroxyiminocyclobutane-1-carboxylate | |
---|---|---|
Source | PubChem | |
URL | https://pubchem.ncbi.nlm.nih.gov | |
Description | Data deposited in or computed by PubChem | |
InChI |
InChI=1S/C7H11NO3/c1-2-11-7(9)5-3-6(4-5)8-10/h5,10H,2-4H2,1H3 | |
Source | PubChem | |
URL | https://pubchem.ncbi.nlm.nih.gov | |
Description | Data deposited in or computed by PubChem | |
InChI Key |
JEGAJOKWFLKGII-UHFFFAOYSA-N | |
Source | PubChem | |
URL | https://pubchem.ncbi.nlm.nih.gov | |
Description | Data deposited in or computed by PubChem | |
Canonical SMILES |
CCOC(=O)C1CC(=NO)C1 | |
Source | PubChem | |
URL | https://pubchem.ncbi.nlm.nih.gov | |
Description | Data deposited in or computed by PubChem | |
Molecular Formula |
C7H11NO3 | |
Source | PubChem | |
URL | https://pubchem.ncbi.nlm.nih.gov | |
Description | Data deposited in or computed by PubChem | |
Molecular Weight |
157.17 g/mol | |
Source | PubChem | |
URL | https://pubchem.ncbi.nlm.nih.gov | |
Description | Data deposited in or computed by PubChem | |
Retrosynthesis Analysis
AI-Powered Synthesis Planning: Our tool employs the Template_relevance Pistachio, Template_relevance Bkms_metabolic, Template_relevance Pistachio_ringbreaker, Template_relevance Reaxys, Template_relevance Reaxys_biocatalysis model, leveraging a vast database of chemical reactions to predict feasible synthetic routes.
One-Step Synthesis Focus: Specifically designed for one-step synthesis, it provides concise and direct routes for your target compounds, streamlining the synthesis process.
Accurate Predictions: Utilizing the extensive PISTACHIO, BKMS_METABOLIC, PISTACHIO_RINGBREAKER, REAXYS, REAXYS_BIOCATALYSIS database, our tool offers high-accuracy predictions, reflecting the latest in chemical research and data.
Strategy Settings
Precursor scoring | Relevance Heuristic |
---|---|
Min. plausibility | 0.01 |
Model | Template_relevance |
Template Set | Pistachio/Bkms_metabolic/Pistachio_ringbreaker/Reaxys/Reaxys_biocatalysis |
Top-N result to add to graph | 6 |
Feasible Synthetic Routes
Disclaimer and Information on In-Vitro Research Products
Please be aware that all articles and product information presented on BenchChem are intended solely for informational purposes. The products available for purchase on BenchChem are specifically designed for in-vitro studies, which are conducted outside of living organisms. In-vitro studies, derived from the Latin term "in glass," involve experiments performed in controlled laboratory settings using cells or tissues. It is important to note that these products are not categorized as medicines or drugs, and they have not received approval from the FDA for the prevention, treatment, or cure of any medical condition, ailment, or disease. We must emphasize that any form of bodily introduction of these products into humans or animals is strictly prohibited by law. It is essential to adhere to these guidelines to ensure compliance with legal and ethical standards in research and experimentation.