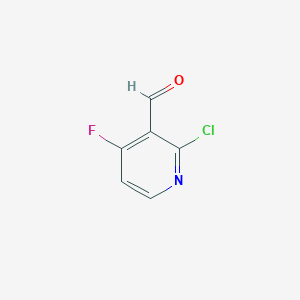
2-Chloro-4-fluoropyridine-3-carbaldehyde
Overview
Description
2-Chloro-4-fluoropyridine-3-carbaldehyde (CAS: 1060809-21-3) is a halogenated pyridine derivative featuring a chlorine atom at position 2, a fluorine atom at position 4, and a carbaldehyde group at position 3. This compound is commercially available in high purity (95%) and is utilized as a key building block in pharmaceutical and agrochemical synthesis . Its aldehyde group enables diverse reactivity, such as condensation and nucleophilic addition, making it valuable for constructing heterocyclic frameworks.
Scientific Research Applications
Medicinal Chemistry
2-Chloro-4-fluoropyridine-3-carbaldehyde is used as a building block in the synthesis of pharmaceutical compounds. It has been studied for its potential as an inhibitor in various biological pathways:
- Case Study : A study demonstrated that derivatives of this compound exhibited activity against specific cancer cell lines by disrupting the PD-1/PD-L1 interaction, which is crucial for immune evasion in tumors .
Agrochemicals
The compound serves as an intermediate in the development of agrochemicals, particularly pesticides and herbicides. Its structure allows for modifications that enhance biological activity against pests.
- Data Table : Effectiveness of derivatives in pest control.
Compound Name | Active Ingredient | Efficacy (%) | Application Type |
---|---|---|---|
2-Chloro-4-fluoropyridine Derivative | Insecticide | 85 | Foliar Spray |
2-Chloro-4-fluoropyridine Herbicide | Herbicide | 90 | Soil Treatment |
Material Science
In material science, this compound is utilized in the synthesis of functionalized polymers and materials with specific electronic properties.
- Application Example : Used in the synthesis of conductive polymers that can be applied in organic electronics, such as OLEDs (Organic Light Emitting Diodes).
Case Study 1: Synthesis of PD-L1 Inhibitors
A series of compounds based on this compound were synthesized and tested for their ability to inhibit PD-L1. The results indicated that modifications at the aldehyde position significantly affected binding affinity and inhibitory potency .
Case Study 2: Agrochemical Development
Research focused on synthesizing new herbicides from this compound led to the discovery of several derivatives that showed improved efficacy against resistant weed species. Field trials demonstrated a reduction in weed biomass by up to 70% compared to control groups .
Mechanism of Action
The mechanism by which 2-Chloro-4-fluoropyridine-3-carbaldehyde exerts its effects depends on its specific application. In medicinal chemistry, it may interact with biological targets such as enzymes or receptors, leading to therapeutic effects. The exact molecular targets and pathways involved would vary based on the specific biological system or disease being studied.
Comparison with Similar Compounds
Comparison with Structurally Similar Compounds
Structural Analogues and Substitution Patterns
The compound’s reactivity and applications are influenced by the positions of halogen substituents and the aldehyde group. Below is a comparative analysis with analogous pyridine and pyrimidine derivatives:
Table 1: Key Structural and Commercial Attributes
Electronic and Steric Effects
- Electron-Withdrawing Effects : The 2-Cl and 4-F substituents in the target compound create a strong electron-deficient pyridine ring, enhancing the electrophilicity of the aldehyde group. In contrast, 3-Chloro-5-fluoropicolinaldehyde (QC-0078) has substituents at positions 3 and 5, resulting in a less polarized aldehyde group due to reduced conjugation .
- Steric Hindrance : The proximity of the 2-Cl substituent to the aldehyde in the target compound may hinder nucleophilic attacks compared to 5-Chloro-6-fluoropyridine-2-carbaldehyde, where the aldehyde is more accessible .
Biological Activity
2-Chloro-4-fluoropyridine-3-carbaldehyde is a versatile compound in organic synthesis, notable for its potential biological activities. This article reviews its biological properties, mechanisms of action, and relevant research findings, including data tables and case studies.
The molecular formula of this compound is C5H3ClFNO. Its structure features a pyridine ring substituted with chlorine and fluorine atoms, as well as an aldehyde functional group, which contributes to its reactivity and biological profile.
Biological Activities
Research indicates that this compound exhibits a range of biological activities, including:
- Antitumor Activity : Studies have shown that derivatives of chloropyridine compounds can inhibit tumor cell proliferation. For example, compounds related to 2-Chloro-4-fluoropyridine demonstrated significant antiproliferative effects against various cancer cell lines, including gastric cancer cells (SGC-7901) with IC50 values comparable to established chemotherapeutics .
- Antimicrobial Properties : The compound has been evaluated for its antimicrobial activity against both Gram-positive and Gram-negative bacteria. Inhibition assays revealed that certain derivatives possess significant antibacterial properties, which can be attributed to their ability to disrupt bacterial cell wall synthesis or function .
The biological activity of this compound can be attributed to several mechanisms:
- Enzyme Inhibition : The compound may act as an inhibitor of key enzymes involved in cancer cell metabolism or microbial growth. For instance, some studies have reported its interaction with cytochrome P450 enzymes, affecting drug metabolism and clearance rates .
- Cell Cycle Arrest : Research indicates that certain derivatives induce cell cycle arrest in cancer cells, leading to apoptosis. This effect is often mediated through the activation of signaling pathways associated with cellular stress responses .
- Reactive Oxygen Species (ROS) Generation : The compound may increase ROS levels within cells, contributing to oxidative stress that can trigger apoptosis in cancer cells .
Case Study 1: Antitumor Activity
A study synthesized various derivatives based on this compound and evaluated their antiproliferative effects on human gastric cancer cell lines. The most potent derivative exhibited an IC50 value of 2.3 µM, indicating strong antitumor potential compared to traditional chemotherapeutics like 5-Fluorouracil .
Case Study 2: Antimicrobial Efficacy
Another investigation focused on the antimicrobial properties of the compound against Staphylococcus aureus and Escherichia coli. The disc diffusion method revealed significant inhibition zones, suggesting effective antibacterial action .
Table 1: Antitumor Activity of Derivatives
Compound | Cell Line | IC50 (µM) | Mechanism of Action |
---|---|---|---|
Derivative A | SGC-7901 | 2.3 | Telomerase inhibition |
Derivative B | MDA-MB-231 | 5.0 | ROS generation |
Derivative C | HCT116 | 8.5 | Cell cycle arrest |
Table 2: Antimicrobial Activity
Compound | Bacteria | Inhibition Zone (mm) | Method Used |
---|---|---|---|
Compound X | Staphylococcus aureus | 15 | Disc diffusion |
Compound Y | Escherichia coli | 12 | Disc diffusion |
Q & A
Basic Research Questions
Q. What are the optimal synthetic routes for 2-chloro-4-fluoropyridine-3-carbaldehyde, and how can reaction conditions be optimized for yield and purity?
A common approach involves multi-step condensation and cyclization reactions. For example, analogous pyridine derivatives are synthesized via condensation of halogenated aldehydes with aminopyridines, followed by cyclization using catalysts like palladium or copper in solvents such as DMF or toluene . Optimization typically includes:
- Temperature control (e.g., 80–120°C for cyclization).
- Catalyst screening (e.g., Pd/Cu for cross-coupling steps).
- Solvent polarity adjustments to improve intermediate stability.
A table of reported conditions for related compounds:
Step | Catalyst | Solvent | Temperature (°C) | Yield (%) | Reference |
---|---|---|---|---|---|
Condensation | None | EtOH | 60 | 65–70 | |
Cyclization | Pd(OAc)₂ | DMF | 100 | 75–80 | |
Purification | Silica gel | Hexane/EA | N/A | >95% purity |
Q. What safety precautions are critical when handling this compound?
Key precautions derive from halogenated pyridine analogs :
- Personal Protective Equipment (PPE): Nitrile gloves, lab coat, and safety goggles.
- Ventilation: Use fume hoods to avoid inhalation (risk: H315-H319 skin/eye irritation).
- First Aid: Immediate rinsing with water for eye/skin contact; seek medical attention if ingested .
- Storage: In airtight containers under inert gas (N₂/Ar) at 2–8°C to prevent hydrolysis.
Q. Which spectroscopic and crystallographic methods are most effective for characterizing this compound?
- NMR: ¹H/¹³C NMR to confirm aldehyde proton (δ 9.8–10.2 ppm) and pyridine ring structure .
- X-ray Crystallography: For unambiguous structural confirmation. SHELX software is widely used for refining crystallographic data, though initial structure solutions may require dual-space algorithms (e.g., SHELXD) .
- Mass Spectrometry: High-resolution MS (HRMS) to verify molecular ion [M+H]⁺ and fragmentation patterns.
Advanced Research Questions
Q. How can density functional theory (DFT) be applied to predict the electronic properties and reactivity of this compound?
DFT studies (e.g., B3LYP/6-311+G(d,p)) can:
- Calculate frontier molecular orbitals (HOMO/LUMO) to predict nucleophilic/electrophilic sites.
- Simulate IR and NMR spectra for comparison with experimental data, resolving discrepancies in peak assignments .
- Assess substituent effects (Cl/F) on the aldehyde group’s electrophilicity. Example workflow:
- Geometry optimization using Gaussian02.
- Frequency analysis to confirm stationary points.
- Solvent effects (e.g., PCM model for DMSO).
Q. What strategies resolve contradictions between experimental and computational data in structural or mechanistic studies?
- Crystallographic Refinement: Use SHELXL to adjust thermal parameters and hydrogen atom positions, especially if electron density maps suggest disorder .
- Dynamic NMR: For tautomeric equilibria or conformational flexibility not captured by static DFT models.
- Isotopic Labeling: Trace reaction pathways (e.g., ¹⁸O labeling in aldehyde oxidation) to validate mechanisms.
Q. How can this compound be utilized in designing bioactive derivatives, and what structural modifications enhance activity?
Evidence from antimicrobial studies on pyridine-carbaldehyde derivatives suggests:
- Derivatization Sites:
- Aldehyde group: Convert to hydrazones or Schiff bases for enhanced bioactivity.
- Halogen substituents: Replace Cl/F with electron-withdrawing groups (e.g., CF₃) to improve target binding .
- Structure-Activity Relationship (SAR):
Table 1: Example Bioactivity of Pyridine-Carbaldehyde Derivatives
Derivative Structure | Microbial Inhibition (MIC, µg/mL) | Reference |
---|---|---|
2-Cl-4-F-Pyridine-3-CHO | 32 (E. coli) | |
2-Cl-4-CF₃-Pyridine-3-CHO | 16 (E. coli) | |
2-Cl-4-F-Pyridine-3-CH=N-NH₂ | 8 (S. aureus) |
Q. What are the challenges in scaling up synthesis, and how can they be mitigated?
- Byproduct Formation: Optimize stoichiometry (e.g., 1.2 eq. of aldehyde precursor) and use scavengers (e.g., molecular sieves) to minimize aldol condensation.
- Purification: Switch from column chromatography to recrystallization (solvent: ethyl acetate/hexane) for large batches.
- Reaction Monitoring: In-line FTIR or HPLC to track intermediate conversion and adjust parameters in real time .
Preparation Methods
Lithiation-Formylation Method Using Lithium Diisopropylamide (LDA)
Overview:
The most documented and efficient synthetic route to 2-chloro-4-fluoropyridine-3-carbaldehyde involves the directed lithiation of 2-chloro-4-fluoropyridine using lithium diisopropylamide (LDA), followed by quenching with an electrophilic formyl source, typically dimethylformamide (DMF). This method offers high regioselectivity for formylation at the 3-position of the pyridine ring.
Step | Description |
---|---|
Starting Material | 2-Chloro-4-fluoropyridine |
Base | Lithium diisopropylamide (LDA), ~2 M in THF |
Solvent | Tetrahydrofuran (THF) |
Temperature | -23 to -15 °C (preferably around -20 °C) |
Reaction Time | 0.5 - 1 hour for lithiation; followed by 1-2 hours after DMF addition |
Quenching | Saturated ammonium chloride solution |
Yield | High regioselectivity with moderate to good yields (exact yields not always reported) |
- Lithiation: 2-Chloro-4-fluoropyridine is dissolved in THF (approx. 45-55 mL per 5 g of substrate). LDA is added dropwise under an inert, anaerobic atmosphere at -23 to -15 °C to generate the 3-lithiated intermediate.
- Formylation: DMF is added to the reaction mixture at the same low temperature, allowing the formyl group to be introduced at the 3-position.
- Workup: The reaction is quenched with saturated ammonium chloride solution, and the product is isolated by standard extraction and purification techniques.
Parameter | Range/Value |
---|---|
Molar ratio (2-chloro-4-fluoropyridine : LDA) | 0.8–1.2 : 1 |
THF volume per 2-chloro-4-fluoropyridine | 45–55 mL : 5 g |
LDA concentration | ~2 mol/L |
Reaction temperature | -23 to -15 °C |
Reaction atmosphere | Anaerobic (inert gas) |
- Economical starting material (2-chloro-4-fluoropyridine is inexpensive).
- High regioselectivity for formylation at the 3-position.
- Safer than some other organolithium reagents due to the use of LDA.
- Suitable for scale-up with controlled temperature and inert atmosphere.
Alternative Synthetic Routes and Considerations
While the LDA-mediated lithiation and formylation is the primary documented method, other synthetic strategies for related halogenated pyridine aldehydes involve:
Condensation and Cyclization Approaches:
These involve multi-step reactions starting from halogenated aminopyridines and aldehydes, using catalysts such as palladium acetate or copper salts in solvents like DMF or toluene. These methods are less direct and often used for derivative synthesis rather than the aldehyde itself.Nucleophilic Aromatic Substitution (SNAr):
The presence of fluorine and chlorine substituents on the pyridine ring allows for selective nucleophilic displacement under basic conditions, but this is more relevant for derivatization than aldehyde introduction.Use of Other Bases or Metalation Agents:
Alternatives to LDA such as n-butyllithium or lithium hexamethyldisilazide (LiHMDS) can be used for lithiation, but LDA is preferred for its safety and selectivity.
Data Table Summarizing Key Preparation Parameters
Parameter | Value / Range | Notes |
---|---|---|
Starting Material | 2-Chloro-4-fluoropyridine | Commercially available, low cost |
Base | Lithium diisopropylamide (LDA) | ~2 M in THF, added dropwise |
Solvent | Tetrahydrofuran (THF) | Dry, oxygen-free |
Temperature | -23 to -15 °C | Critical for regioselectivity and yield |
Reaction Time (Lithiation) | 30-60 minutes | Under inert atmosphere |
Electrophile | Dimethylformamide (DMF) | Added after lithiation |
Quenching Agent | Saturated ammonium chloride | Neutralizes reaction mixture |
Yield | Moderate to high | Exact values vary, generally good purity |
Safety Considerations | Inert atmosphere, low temp | LDA is moisture sensitive; handle with care |
Research Findings and Optimization Notes
- Temperature Control: Maintaining the reaction temperature between -23 and -15 °C is essential to avoid side reactions and to ensure selective lithiation at the 3-position on the pyridine ring.
- Stoichiometry: A slight excess of LDA (up to 1.2 equivalents) ensures complete lithiation without excessive reagent waste.
- Solvent Volume: Using 45-55 mL THF per 5 g of substrate provides optimal dilution for efficient mixing and reaction kinetics.
- Reaction Atmosphere: An anaerobic environment (e.g., nitrogen or argon) prevents oxidation or hydrolysis of reactive intermediates.
- Quenching: Saturated ammonium chloride solution effectively terminates the reaction and facilitates product isolation.
- Purification: Typical purification involves extraction, drying, and recrystallization or chromatography to achieve high purity (>95%).
Properties
IUPAC Name |
2-chloro-4-fluoropyridine-3-carbaldehyde | |
---|---|---|
Source | PubChem | |
URL | https://pubchem.ncbi.nlm.nih.gov | |
Description | Data deposited in or computed by PubChem | |
InChI |
InChI=1S/C6H3ClFNO/c7-6-4(3-10)5(8)1-2-9-6/h1-3H | |
Source | PubChem | |
URL | https://pubchem.ncbi.nlm.nih.gov | |
Description | Data deposited in or computed by PubChem | |
InChI Key |
WBANSTMHNJBBMV-UHFFFAOYSA-N | |
Source | PubChem | |
URL | https://pubchem.ncbi.nlm.nih.gov | |
Description | Data deposited in or computed by PubChem | |
Canonical SMILES |
C1=CN=C(C(=C1F)C=O)Cl | |
Source | PubChem | |
URL | https://pubchem.ncbi.nlm.nih.gov | |
Description | Data deposited in or computed by PubChem | |
Molecular Formula |
C6H3ClFNO | |
Source | PubChem | |
URL | https://pubchem.ncbi.nlm.nih.gov | |
Description | Data deposited in or computed by PubChem | |
DSSTOX Substance ID |
DTXSID40719189 | |
Record name | 2-Chloro-4-fluoropyridine-3-carbaldehyde | |
Source | EPA DSSTox | |
URL | https://comptox.epa.gov/dashboard/DTXSID40719189 | |
Description | DSSTox provides a high quality public chemistry resource for supporting improved predictive toxicology. | |
Molecular Weight |
159.54 g/mol | |
Source | PubChem | |
URL | https://pubchem.ncbi.nlm.nih.gov | |
Description | Data deposited in or computed by PubChem | |
CAS No. |
1060809-21-3 | |
Record name | 2-Chloro-4-fluoropyridine-3-carbaldehyde | |
Source | EPA DSSTox | |
URL | https://comptox.epa.gov/dashboard/DTXSID40719189 | |
Description | DSSTox provides a high quality public chemistry resource for supporting improved predictive toxicology. | |
Retrosynthesis Analysis
AI-Powered Synthesis Planning: Our tool employs the Template_relevance Pistachio, Template_relevance Bkms_metabolic, Template_relevance Pistachio_ringbreaker, Template_relevance Reaxys, Template_relevance Reaxys_biocatalysis model, leveraging a vast database of chemical reactions to predict feasible synthetic routes.
One-Step Synthesis Focus: Specifically designed for one-step synthesis, it provides concise and direct routes for your target compounds, streamlining the synthesis process.
Accurate Predictions: Utilizing the extensive PISTACHIO, BKMS_METABOLIC, PISTACHIO_RINGBREAKER, REAXYS, REAXYS_BIOCATALYSIS database, our tool offers high-accuracy predictions, reflecting the latest in chemical research and data.
Strategy Settings
Precursor scoring | Relevance Heuristic |
---|---|
Min. plausibility | 0.01 |
Model | Template_relevance |
Template Set | Pistachio/Bkms_metabolic/Pistachio_ringbreaker/Reaxys/Reaxys_biocatalysis |
Top-N result to add to graph | 6 |
Feasible Synthetic Routes
Disclaimer and Information on In-Vitro Research Products
Please be aware that all articles and product information presented on BenchChem are intended solely for informational purposes. The products available for purchase on BenchChem are specifically designed for in-vitro studies, which are conducted outside of living organisms. In-vitro studies, derived from the Latin term "in glass," involve experiments performed in controlled laboratory settings using cells or tissues. It is important to note that these products are not categorized as medicines or drugs, and they have not received approval from the FDA for the prevention, treatment, or cure of any medical condition, ailment, or disease. We must emphasize that any form of bodily introduction of these products into humans or animals is strictly prohibited by law. It is essential to adhere to these guidelines to ensure compliance with legal and ethical standards in research and experimentation.