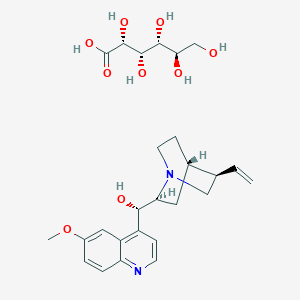
Quinidine gluconate
- Click on QUICK INQUIRY to receive a quote from our team of experts.
- With the quality product at a COMPETITIVE price, you can focus more on your research.
Overview
Description
Quinidine gluconate is a pharmaceutical compound derived from quinidine, which is a D-isomer of quinine. Quinine is naturally found in the bark of the Cinchona tree and similar plant species. This compound is primarily used as an antiarrhythmic agent to treat various types of irregular heartbeats, including atrial fibrillation and ventricular arrhythmias . It is also known for its antimalarial properties .
Mechanism of Action
Target of Action
Quinidine gluconate primarily targets sodium channels in cardiac muscle and Purkinje fibers . These channels play a crucial role in the electrical activity of the heart, regulating the flow of sodium ions into cardiac cells, which is essential for the initiation and propagation of the cardiac action potential .
Mode of Action
This compound is a Class Ia antiarrhythmic agent . It works by blocking the rapid sodium channel (I Na), which decreases the phase zero of rapid depolarization of the action potential . This action prolongs the cellular action potential, thereby decreasing myocardial excitability and conduction velocity .
Biochemical Pathways
The antiarrhythmic actions of this compound are mediated through its effects on sodium channels in Purkinje fibers . By blocking these channels, this compound disrupts the normal flow of sodium ions, which in turn affects the electrical activity of the heart. This can help to restore normal sinus rhythm and treat conditions such as atrial fibrillation and flutter .
Pharmacokinetics
The absolute bioavailability of quinidine from this compound is 70 to 80% . This means that when the drug is administered orally, about 70 to 80% of the dose reaches the systemic circulation. The less-than-complete bioavailability is a result of first-pass metabolism in the liver .
Result of Action
The primary result of this compound’s action is the restoration of normal sinus rhythm in the heart . It is moderately efficacious in the acute conversion of atrial fibrillation to normal sinus rhythm . It’s important to note that this compound can have side effects, including an increased risk of mortality .
Action Environment
The action of this compound can be influenced by various environmental factors. For example, the presence of other medications can affect its efficacy and safety. This compound is known to interact with a large number of other medications in potentially dangerous or unpredictable ways .
Biochemical Analysis
Biochemical Properties
Quinidine gluconate plays a significant role in biochemical reactions, particularly in the cardiovascular system. It interacts with several enzymes, proteins, and other biomolecules. One of the primary interactions is with sodium and potassium channels in cardiac cells. This compound blocks these channels, which prolongs the action potential and refractory period of the cardiac cells . This action helps to stabilize the heart rhythm and prevent arrhythmias. Additionally, this compound has been shown to interact with cytochrome P450 enzymes in the liver, affecting its metabolism and bioavailability .
Cellular Effects
This compound exerts various effects on different types of cells and cellular processes. In cardiac cells, it influences cell function by blocking sodium and potassium channels, which affects the action potential duration and refractory period . This action helps to stabilize the heart rhythm and prevent arrhythmias. This compound also impacts cell signaling pathways, gene expression, and cellular metabolism. For example, it has been shown to inhibit the activity of certain enzymes involved in cellular metabolism, leading to changes in metabolite levels and metabolic flux .
Molecular Mechanism
The molecular mechanism of this compound involves its interaction with sodium and potassium channels in cardiac cells. By blocking these channels, this compound prolongs the action potential and refractory period, which helps to stabilize the heart rhythm . Additionally, this compound can inhibit the activity of certain enzymes, such as cytochrome P450 enzymes, which affects its metabolism and bioavailability . These interactions at the molecular level contribute to the overall therapeutic effects of this compound.
Temporal Effects in Laboratory Settings
In laboratory settings, the effects of this compound can change over time. The stability and degradation of the compound are important factors that influence its long-term effects on cellular function. Studies have shown that this compound is relatively stable, but it can degrade over time, leading to changes in its efficacy and safety . Long-term effects on cellular function have been observed in both in vitro and in vivo studies, with some studies reporting changes in cell signaling pathways, gene expression, and cellular metabolism over time .
Dosage Effects in Animal Models
The effects of this compound can vary with different dosages in animal models. Studies have shown that there are threshold effects, where low doses of this compound can have therapeutic effects, while higher doses can lead to toxic or adverse effects . For example, in dogs, dosages of 6–20 mg/kg have been used to treat arrhythmias, but higher doses can lead to toxicity and adverse effects such as gastrointestinal disturbances and cardiac arrhythmias . These dosage-dependent effects highlight the importance of careful dosing and monitoring in clinical settings.
Metabolic Pathways
This compound is involved in several metabolic pathways, primarily in the liver. It is metabolized by cytochrome P450 enzymes, which convert it into various metabolites, including 3-hydroxy-quinidine . This metabolite has been shown to have similar, but less potent, antiarrhythmic effects compared to this compound . The metabolism of this compound can affect its bioavailability and therapeutic efficacy, making it important to consider these pathways in clinical settings.
Transport and Distribution
The transport and distribution of this compound within cells and tissues are important factors that influence its therapeutic effects. This compound is transported across cell membranes by various transporters, including P-glycoprotein . Once inside the cells, it can interact with various binding proteins, which affect its localization and accumulation. The distribution of this compound within tissues can also influence its therapeutic effects, with higher concentrations in cardiac tissues leading to more pronounced antiarrhythmic effects .
Subcellular Localization
The subcellular localization of this compound can affect its activity and function. This compound is primarily localized in the cytoplasm of cardiac cells, where it interacts with sodium and potassium channels . This localization is important for its antiarrhythmic effects, as it allows this compound to effectively block these channels and stabilize the heart rhythm. Additionally, this compound can undergo post-translational modifications that direct it to specific compartments or organelles within the cells .
Preparation Methods
Synthetic Routes and Reaction Conditions: Quinidine gluconate can be synthesized from quinine. The process involves the conversion of quinine to quinidine, followed by the formation of the gluconate salt. The reaction typically involves the use of glacial acetic acid and phenylhydrazine hydrochloride, followed by heating in a water bath .
Industrial Production Methods: Industrial production of this compound involves the extraction of quinine from the bark of the Cinchona tree, followed by its conversion to quinidine. The quinidine is then reacted with gluconic acid to form this compound. The final product is purified using chromatographic techniques to ensure high purity and quality .
Chemical Reactions Analysis
Types of Reactions: Quinidine gluconate undergoes various chemical reactions, including:
Oxidation: Quinidine can be oxidized to form quinidine N-oxide.
Reduction: Quinidine can be reduced to form dihydroquinidine.
Substitution: Quinidine can undergo substitution reactions, particularly at the methoxy group.
Common Reagents and Conditions:
Oxidation: Common oxidizing agents include hydrogen peroxide and peracids.
Reduction: Reducing agents such as sodium borohydride or lithium aluminum hydride are used.
Substitution: Substitution reactions often involve nucleophiles like halides or amines under basic conditions.
Major Products:
Oxidation: Quinidine N-oxide.
Reduction: Dihydroquinidine.
Substitution: Various substituted quinidine derivatives depending on the nucleophile used.
Scientific Research Applications
Quinidine gluconate has a wide range of applications in scientific research:
Chemistry: Used as a chiral catalyst in asymmetric synthesis.
Biology: Studied for its effects on ion channels and cellular action potentials.
Medicine: Used to treat cardiac arrhythmias and as an antimalarial agent.
Industry: Employed in the production of other pharmaceutical compounds and as a reference standard in analytical chemistry
Comparison with Similar Compounds
Quinine: An antimalarial agent with similar chemical structure but different pharmacological properties.
Procainamide: Another class Ia antiarrhythmic agent with similar effects on cardiac action potentials.
Disopyramide: A class Ia antiarrhythmic agent used to treat ventricular arrhythmias.
Uniqueness: Quinidine gluconate is unique due to its dual role as an antiarrhythmic and antimalarial agent. It has a higher efficacy in converting atrial fibrillation to normal sinus rhythm compared to other class Ia antiarrhythmics. Additionally, its gluconate form provides better bioavailability and prolonged action .
Properties
CAS No. |
7054-25-3 |
---|---|
Molecular Formula |
C26H36N2O9 |
Molecular Weight |
520.6 g/mol |
IUPAC Name |
(S)-[(4S,5R)-5-ethenyl-1-azabicyclo[2.2.2]octan-2-yl]-(6-methoxyquinolin-4-yl)methanol;(2R,3S,4R,5R)-2,3,4,5,6-pentahydroxyhexanoic acid |
InChI |
InChI=1S/C20H24N2O2.C6H12O7/c1-3-13-12-22-9-7-14(13)10-19(22)20(23)16-6-8-21-18-5-4-15(24-2)11-17(16)18;7-1-2(8)3(9)4(10)5(11)6(12)13/h3-6,8,11,13-14,19-20,23H,1,7,9-10,12H2,2H3;2-5,7-11H,1H2,(H,12,13)/t13-,14-,19?,20-;2-,3-,4+,5-/m01/s1 |
InChI Key |
XHKUDCCTVQUHJQ-OSPZJNBRSA-N |
SMILES |
COC1=CC2=C(C=CN=C2C=C1)C(C3CC4CCN3CC4C=C)O.C(C(C(C(C(C(=O)O)O)O)O)O)O |
Isomeric SMILES |
COC1=CC2=C(C=CN=C2C=C1)[C@@H](C3C[C@@H]4CCN3C[C@@H]4C=C)O.C([C@H]([C@H]([C@@H]([C@H](C(=O)O)O)O)O)O)O |
Canonical SMILES |
COC1=CC2=C(C=CN=C2C=C1)C(C3CC4CCN3CC4C=C)O.C(C(C(C(C(C(=O)O)O)O)O)O)O |
Pictograms |
Acute Toxic; Irritant |
Synonyms |
D-Gluconic Acid compd. with Quinidine (1:1); Quinidine Gluconate; (9S)-6’-Methoxy-cinchonan-9-ol Mono-D-gluconate (Salt); Quinidine, mono-D-Gluconate (Salt); Dura-Tab; Duraquin; Gluquinate; Quinaglute; Quinate |
Origin of Product |
United States |
Retrosynthesis Analysis
AI-Powered Synthesis Planning: Our tool employs the Template_relevance Pistachio, Template_relevance Bkms_metabolic, Template_relevance Pistachio_ringbreaker, Template_relevance Reaxys, Template_relevance Reaxys_biocatalysis model, leveraging a vast database of chemical reactions to predict feasible synthetic routes.
One-Step Synthesis Focus: Specifically designed for one-step synthesis, it provides concise and direct routes for your target compounds, streamlining the synthesis process.
Accurate Predictions: Utilizing the extensive PISTACHIO, BKMS_METABOLIC, PISTACHIO_RINGBREAKER, REAXYS, REAXYS_BIOCATALYSIS database, our tool offers high-accuracy predictions, reflecting the latest in chemical research and data.
Strategy Settings
Precursor scoring | Relevance Heuristic |
---|---|
Min. plausibility | 0.01 |
Model | Template_relevance |
Template Set | Pistachio/Bkms_metabolic/Pistachio_ringbreaker/Reaxys/Reaxys_biocatalysis |
Top-N result to add to graph | 6 |
Feasible Synthetic Routes
Disclaimer and Information on In-Vitro Research Products
Please be aware that all articles and product information presented on BenchChem are intended solely for informational purposes. The products available for purchase on BenchChem are specifically designed for in-vitro studies, which are conducted outside of living organisms. In-vitro studies, derived from the Latin term "in glass," involve experiments performed in controlled laboratory settings using cells or tissues. It is important to note that these products are not categorized as medicines or drugs, and they have not received approval from the FDA for the prevention, treatment, or cure of any medical condition, ailment, or disease. We must emphasize that any form of bodily introduction of these products into humans or animals is strictly prohibited by law. It is essential to adhere to these guidelines to ensure compliance with legal and ethical standards in research and experimentation.