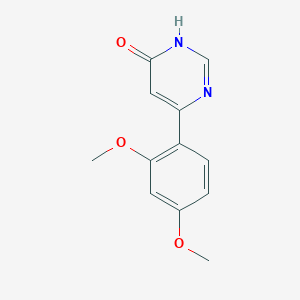
6-(2,4-Dimethoxyphenyl)pyrimidin-4-ol
Overview
Description
6-(2,4-Dimethoxyphenyl)pyrimidin-4-ol is a chemical compound characterized by its unique structure, which includes a pyrimidin-4-ol core and a 2,4-dimethoxyphenyl group
Biochemical Analysis
Biochemical Properties
6-(2,4-Dimethoxyphenyl)pyrimidin-4-ol plays a significant role in biochemical reactions, particularly in the inhibition of specific enzymes. One of the key enzymes it interacts with is Aurora kinase A (AURKA). This compound has been shown to selectively inhibit AURKA activity, which is crucial for cell cycle regulation and mitosis . The interaction between this compound and AURKA involves binding to the active site of the enzyme, thereby preventing its phosphorylation and subsequent activation. This inhibition leads to the disruption of cell division and has potential implications for cancer therapy.
Cellular Effects
The effects of this compound on various types of cells and cellular processes are profound. In cancer cells, this compound induces cell cycle arrest at the G2/M phase, leading to the accumulation of cells in this phase and preventing their progression to mitosis . Additionally, this compound triggers apoptotic cell death through the activation of caspase-3 and caspase-7, as well as the cleavage of poly (ADP-ribose) polymerase (PARP) . These effects are mediated through the inhibition of AURKA, which plays a pivotal role in cell division and survival.
Molecular Mechanism
At the molecular level, this compound exerts its effects through the inhibition of AURKA. The compound binds to the ATP-binding pocket of AURKA, thereby preventing the phosphorylation of its substrates . This inhibition disrupts the normal function of AURKA in promoting cell division and leads to cell cycle arrest and apoptosis. Additionally, the binding of this compound to AURKA has been confirmed through in silico docking studies, which demonstrate a strong binding affinity and stability of the compound-enzyme complex .
Temporal Effects in Laboratory Settings
In laboratory settings, the effects of this compound have been observed to change over time. The compound exhibits stability under standard laboratory conditions, with minimal degradation over extended periods . Long-term studies have shown that continuous exposure to this compound leads to sustained inhibition of AURKA activity and prolonged cell cycle arrest . The compound’s effects on cellular function may diminish over time due to adaptive cellular responses and potential development of resistance mechanisms.
Dosage Effects in Animal Models
The effects of this compound vary with different dosages in animal models. At low doses, the compound effectively inhibits AURKA activity and induces cell cycle arrest without causing significant toxicity . At higher doses, this compound may exhibit toxic effects, including hepatotoxicity and nephrotoxicity . These adverse effects are dose-dependent and highlight the importance of optimizing the dosage for therapeutic applications to minimize toxicity while maximizing efficacy.
Metabolic Pathways
This compound is involved in several metabolic pathways, primarily through its interactions with enzymes such as flavin-containing monooxygenases (FMOs) . The compound undergoes oxidative metabolism, leading to the formation of various metabolites . These metabolic transformations can influence the compound’s activity and stability, as well as its overall pharmacokinetic profile. The involvement of FMOs in the metabolism of this compound underscores the importance of understanding its metabolic pathways for optimizing its therapeutic potential.
Transport and Distribution
Within cells and tissues, this compound is transported and distributed through interactions with specific transporters and binding proteins . The compound’s distribution is influenced by its physicochemical properties, such as lipophilicity and molecular size. Studies have shown that this compound accumulates in certain tissues, including the liver and kidneys, where it exerts its biochemical effects . The transport and distribution of this compound are critical factors in determining its efficacy and safety in therapeutic applications.
Subcellular Localization
The subcellular localization of this compound is primarily within the cytoplasm, where it interacts with its target enzyme, AURKA . The compound’s localization is facilitated by its ability to diffuse across cellular membranes and reach the cytoplasmic compartment. Additionally, post-translational modifications and targeting signals may influence the precise localization and activity of this compound within specific cellular compartments . Understanding the subcellular localization of this compound is essential for elucidating its mechanism of action and optimizing its therapeutic potential.
Preparation Methods
Synthetic Routes and Reaction Conditions: The synthesis of 6-(2,4-Dimethoxyphenyl)pyrimidin-4-ol typically involves a multi-step reaction process. One common method is the Biginelli reaction, which involves the condensation of an aldehyde (such as 2,4-dimethoxybenzaldehyde), a β-keto ester (such as ethyl acetoacetate), and urea in the presence of a catalyst (such as p-toluenesulfonic acid) under reflux conditions.
Industrial Production Methods: In an industrial setting, the synthesis of this compound may involve large-scale reactions with optimized conditions to ensure high yield and purity. The use of continuous flow reactors and automated systems can enhance the efficiency and scalability of the production process.
Chemical Reactions Analysis
Types of Reactions: 6-(2,4-Dimethoxyphenyl)pyrimidin-4-ol can undergo various chemical reactions, including oxidation, reduction, and substitution reactions.
Common Reagents and Conditions:
Oxidation: Common oxidizing agents include potassium permanganate (KMnO₄) and hydrogen peroxide (H₂O₂).
Reduction: Reducing agents such as lithium aluminum hydride (LiAlH₄) and sodium borohydride (NaBH₄) can be used.
Substitution: Nucleophilic substitution reactions can be carried out using various nucleophiles in the presence of a suitable solvent.
Major Products Formed:
Oxidation: Formation of corresponding carboxylic acids or ketones.
Reduction: Formation of corresponding alcohols or amines.
Substitution: Formation of various substituted pyrimidin-4-ol derivatives.
Scientific Research Applications
Chemistry: In chemistry, 6-(2,4-Dimethoxyphenyl)pyrimidin-4-ol is used as a building block for the synthesis of more complex molecules. Its unique structure makes it a valuable intermediate in organic synthesis.
Biology: This compound has shown potential biological activities, including antiviral, anti-inflammatory, and anticancer properties. It can be used in the development of new therapeutic agents.
Medicine: In the medical field, this compound is being investigated for its potential use in drug discovery and development. Its ability to interact with various molecular targets makes it a promising candidate for pharmaceutical applications.
Industry: In industry, this compound can be used in the production of dyes, pigments, and other chemical products. Its versatility and reactivity make it a valuable component in various industrial processes.
Mechanism of Action
The mechanism by which 6-(2,4-Dimethoxyphenyl)pyrimidin-4-ol exerts its effects involves its interaction with specific molecular targets and pathways. For example, in biological systems, it may bind to receptors or enzymes, leading to the modulation of biological processes. The exact mechanism can vary depending on the specific application and context.
Comparison with Similar Compounds
2-(2,4-Dimethoxyphenyl)propan-2-ol
Ethyl (E)-4-(2,4-Dimethoxyphenyl)-6-(2,4-dimethoxystyryl)-2-oxo-1,2,3,4-tetrahydropyrimidine-5-carboxylate
Uniqueness: 6-(2,4-Dimethoxyphenyl)pyrimidin-4-ol stands out due to its specific structural features and its potential applications in various fields. While similar compounds may share some properties, the unique combination of the pyrimidin-4-ol core and the 2,4-dimethoxyphenyl group gives it distinct advantages in terms of reactivity and biological activity.
Properties
IUPAC Name |
4-(2,4-dimethoxyphenyl)-1H-pyrimidin-6-one | |
---|---|---|
Source | PubChem | |
URL | https://pubchem.ncbi.nlm.nih.gov | |
Description | Data deposited in or computed by PubChem | |
InChI |
InChI=1S/C12H12N2O3/c1-16-8-3-4-9(11(5-8)17-2)10-6-12(15)14-7-13-10/h3-7H,1-2H3,(H,13,14,15) | |
Source | PubChem | |
URL | https://pubchem.ncbi.nlm.nih.gov | |
Description | Data deposited in or computed by PubChem | |
InChI Key |
IAXOWGBXDZFTHB-UHFFFAOYSA-N | |
Source | PubChem | |
URL | https://pubchem.ncbi.nlm.nih.gov | |
Description | Data deposited in or computed by PubChem | |
Canonical SMILES |
COC1=CC(=C(C=C1)C2=CC(=O)NC=N2)OC | |
Source | PubChem | |
URL | https://pubchem.ncbi.nlm.nih.gov | |
Description | Data deposited in or computed by PubChem | |
Molecular Formula |
C12H12N2O3 | |
Source | PubChem | |
URL | https://pubchem.ncbi.nlm.nih.gov | |
Description | Data deposited in or computed by PubChem | |
Molecular Weight |
232.23 g/mol | |
Source | PubChem | |
URL | https://pubchem.ncbi.nlm.nih.gov | |
Description | Data deposited in or computed by PubChem | |
Retrosynthesis Analysis
AI-Powered Synthesis Planning: Our tool employs the Template_relevance Pistachio, Template_relevance Bkms_metabolic, Template_relevance Pistachio_ringbreaker, Template_relevance Reaxys, Template_relevance Reaxys_biocatalysis model, leveraging a vast database of chemical reactions to predict feasible synthetic routes.
One-Step Synthesis Focus: Specifically designed for one-step synthesis, it provides concise and direct routes for your target compounds, streamlining the synthesis process.
Accurate Predictions: Utilizing the extensive PISTACHIO, BKMS_METABOLIC, PISTACHIO_RINGBREAKER, REAXYS, REAXYS_BIOCATALYSIS database, our tool offers high-accuracy predictions, reflecting the latest in chemical research and data.
Strategy Settings
Precursor scoring | Relevance Heuristic |
---|---|
Min. plausibility | 0.01 |
Model | Template_relevance |
Template Set | Pistachio/Bkms_metabolic/Pistachio_ringbreaker/Reaxys/Reaxys_biocatalysis |
Top-N result to add to graph | 6 |
Feasible Synthetic Routes
Disclaimer and Information on In-Vitro Research Products
Please be aware that all articles and product information presented on BenchChem are intended solely for informational purposes. The products available for purchase on BenchChem are specifically designed for in-vitro studies, which are conducted outside of living organisms. In-vitro studies, derived from the Latin term "in glass," involve experiments performed in controlled laboratory settings using cells or tissues. It is important to note that these products are not categorized as medicines or drugs, and they have not received approval from the FDA for the prevention, treatment, or cure of any medical condition, ailment, or disease. We must emphasize that any form of bodily introduction of these products into humans or animals is strictly prohibited by law. It is essential to adhere to these guidelines to ensure compliance with legal and ethical standards in research and experimentation.