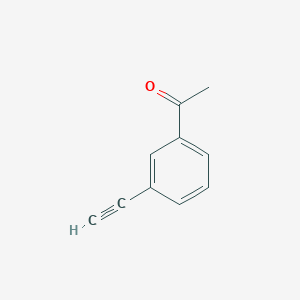
1-(3-Ethynylphenyl)ethanone
Overview
Description
1-(3-Ethynylphenyl)ethanone is a phenyl ethanone derivative characterized by an ethynyl (-C≡CH) substituent at the meta position of the aromatic ring. This compound belongs to a broader class of acetophenone derivatives, which are widely studied for their diverse applications in medicinal chemistry, material science, and synthetic organic chemistry.
Preparation Methods
Sonogashira Cross-Coupling as a Primary Synthetic Route
Catalytic System and Reaction Parameters
The Sonogashira coupling reaction is the most widely reported method for synthesizing 1-(3-Ethynylphenyl)ethanone. This palladium-catalyzed process couples a halogenated phenyl ethanone precursor (e.g., 3-iodoacetophenone) with a terminal alkyne. Critical components include:
-
Solvents : Tetrahydrofuran (THF) or dimethylformamide (DMF)/acetonitrile (CH₃CN) mixtures .
-
Base : Triethylamine (Et₃N) or inorganic bases (e.g., K₂CO₃) .
Reactions proceed under nitrogen at room temperature, achieving complete conversion within 16–24 hours . For example, coupling 3-iodoacetophenone with trimethylsilylacetylene in THF/Et₃N yields 1-(3-((trimethylsilyl)ethynyl)phenyl)ethanone, which is desilylated using K₂CO₃ in methanol to furnish the final product .
Table 1: Comparison of Sonogashira Coupling Conditions
Parameter | Method A | Method B |
---|---|---|
Catalyst | PdCl₂(PPh₃)₂/CuI | Pd(PPh₃)₂Cl₂/CuI |
Solvent | THF | DMF/CH₃CN (1:2) |
Temperature | 25°C | 25°C |
Reaction Time | 16 h | 24 h |
Yield | 68% | 74–80% |
Purity (HPLC) | >99% | >99.9% |
Substrate Scope and Limitations
The meta-substitution pattern in 3-iodoacetophenone presents challenges due to steric hindrance, often necessitating higher catalyst loadings compared to para-substituted analogs . Ethynyltrimethylsilane is preferred over acetylene gas for safety and handling, though desilylation adds an extra step .
Alternative Synthetic Pathways
Halogenation-Alkyne Substitution Sequences
Brominated intermediates offer an alternative route. For instance, 1-(3-bromophenyl)ethanone undergoes coupling with ethynylmagnesium bromide in the presence of NiCl₂(PPh₃)₂, though yields are modest (50–55%) . This method is less favored due to competing side reactions (e.g., Glaser coupling) .
Industrial-Scale Production Considerations
Patent literature describes continuous flow reactors for analogous compounds, emphasizing:
-
Solvent Optimization : Chloroform or methylene chloride reduces side-product formation .
-
Purification : Crystallization from isopropyl alcohol achieves >99.9% purity, avoiding chromatography .
-
Catalyst Recycling : Immobilized palladium catalysts reduce costs in large-scale runs .
Critical Analysis of Reaction Work-Up and Purification
Quenching and Isolation
Post-reaction mixtures are typically quenched in ice-cold sodium bicarbonate to neutralize acidic byproducts . Organic layers (e.g., ethyl acetate) are dried over MgSO₄ and concentrated under reduced pressure .
Chromatography vs. Crystallization
Chemical Reactions Analysis
Types of Reactions: 1-(3-Ethynylphenyl)ethanone undergoes various chemical reactions, including:
Oxidation: The compound can be oxidized to form corresponding carboxylic acids or other oxidized derivatives.
Reduction: Reduction reactions can convert the ethanone group to an alcohol or other reduced forms.
Substitution: The ethynyl group can participate in substitution reactions, leading to the formation of various substituted derivatives.
Common Reagents and Conditions:
Oxidation: Common oxidizing agents include potassium permanganate and chromium trioxide.
Reduction: Reducing agents such as sodium borohydride or lithium aluminum hydride are often used.
Substitution: Substitution reactions may involve reagents like halogens, acids, or bases under appropriate conditions.
Major Products:
Oxidation: Carboxylic acids or ketones.
Reduction: Alcohols or alkanes.
Substitution: Various substituted phenyl ethanone derivatives.
Scientific Research Applications
Organic Synthesis
1-(3-Ethynylphenyl)ethanone serves as a versatile building block in organic synthesis. The ethynyl group allows for further chain extension or cyclization reactions, making it valuable in creating more complex organic molecules. Its unique structure can facilitate various transformations, including oxidation and reduction reactions.
Material Science
In industrial applications, this compound is utilized in the production of specialty chemicals and materials. Its unique reactivity makes it suitable for developing new materials with tailored properties for specific applications.
Case Study 1: Anticancer Activity
A study explored the synthesis and biological evaluation of compounds related to this compound as potential EGFR inhibitors. The results demonstrated that certain derivatives exhibited significant antiproliferative effects against EGFR-overexpressing tumor cell lines, suggesting that compounds with similar structures could lead to novel anticancer therapies .
Case Study 2: Synthesis Methodology
Research on synthetic methodologies highlighted the efficiency of using continuous flow reactors for producing this compound on an industrial scale. Optimized reaction conditions improved yield and efficiency while minimizing by-products. This advancement indicates a growing interest in scaling up production for broader applications.
Mechanism of Action
The mechanism of action of 1-(3-Ethynylphenyl)ethanone involves its interaction with various molecular targets. The ethynyl group can participate in π-π interactions, while the ethanone group can form hydrogen bonds with biological molecules. These interactions can influence the compound’s reactivity and biological activity.
Comparison with Similar Compounds
The following analysis compares 1-(3-Ethynylphenyl)ethanone with structurally related phenyl ethanone derivatives, focusing on substituent effects, biological activity, and physicochemical properties.
2.1.1. Hydroxyl and Methoxy Substituents
- Example Compounds: 1-(2,5-Dihydroxy-4-methoxyphenyl)ethanone (Compound 8) 1-(2,3-Dihydroxy-4-methoxyphenyl)ethanone (Compound 9)
- Activity : These compounds exhibit potent α-glucosidase inhibitory activity, with IC₅₀ values correlating with the number of hydroxyl groups. Compound 8 (two hydroxyl groups) showed stronger inhibition than Compound 9 (three hydroxyl groups), suggesting steric or electronic factors may modulate activity .
- Key Insight: Hydroxyl groups enhance hydrogen bonding with enzyme active sites, but excessive substitution may reduce efficacy.
2.1.2. Nitro Substituents
- Example Compound: 1-(3-Nitrophenyl)ethanone
- Activity: Nitro groups are strong electron-withdrawing substituents.
- Key Insight: The ethynyl group’s linear geometry and sp hybridization may offer similar electronic effects to nitro groups but with reduced steric hindrance, enabling novel interactions in catalytic or binding applications.
2.1.3. Methyl Substituents
- Example Compound: 1-(3-Methylphenyl)ethanone
- Activity : Used primarily in structural studies (e.g., NMR analysis), this compound’s methyl group increases lipophilicity, which could enhance membrane permeability in drug design .
Heterocyclic and Indolyl Derivatives
- Example Compounds: 1-(5-Nitro-1H-indol-3-yl)-2-((4-nitrophenyl)thio)ethanone (antiplasmodial activity, pIC₅₀ = 8.2129) 1-(Benzofuran-2-yl)-2-(1H-1,2,4-triazol-1-yl)ethanone (antifungal activity)
- Activity : Indolyl and benzofuran derivatives exhibit enhanced activity due to planar aromatic systems enabling π-π stacking and hydrophobic interactions .
Halogenated Derivatives
- Example Compound: 1-(3-Bromophenyl)ethanone
- Application : Used as an intermediate in Suzuki-Miyaura couplings to synthesize biaryl compounds .
- Key Insight : The ethynyl group’s smaller size and higher reactivity compared to bromo substituents could streamline cross-coupling reactions or enable alkyne-specific transformations (e.g., click chemistry).
Physicochemical Properties
Selected thermal and solubility properties of phenyl ethanones are summarized below:
Molecular Docking and Binding Affinity
- Hydroxylated Derivatives : Compounds 8 and 9 bind α-glucosidase with energies of −0.87 and −0.92 kcal/mol, respectively, outperforming acarbose (−0.24 kcal/mol) .
Biological Activity
1-(3-Ethynylphenyl)ethanone, also known as a phenyl ethanone derivative, is a compound of significant interest due to its diverse biological activities. This article explores its mechanisms of action, biological effects, and relevant research findings.
Chemical Structure and Properties
This compound features an ethynyl group attached to a phenyl ring, which influences its reactivity and interactions with biological targets. The compound can participate in π-π interactions and form hydrogen bonds due to its functional groups, making it suitable for various chemical reactions, including nucleophilic addition and metal complex formation.
The biological activity of this compound is attributed to its interaction with multiple molecular targets. The ethynyl group enhances its ability to engage in specific biochemical pathways, influencing cellular functions such as apoptosis and cell proliferation. Notably, the compound has been shown to exhibit anticancer properties by inhibiting tumor growth through various mechanisms:
- Inhibition of Cell Proliferation : Studies indicate that this compound can inhibit the growth of cancer cells in vitro, particularly in melanoma models. The compound's effectiveness appears to be dose-dependent.
- Induction of Apoptosis : The compound may induce apoptosis in cancer cells by activating specific signaling pathways that lead to programmed cell death .
- Interaction with Enzymes : It has been utilized in studies assessing enzyme interactions, which are crucial for understanding metabolic pathways and drug development.
Anticancer Activity
This compound has demonstrated significant anticancer activity across various studies:
Neuroprotective Effects
Preclinical studies suggest that this compound may also possess neuroprotective properties:
- Mechanism : It is believed to block inflammatory pathways and protect neuronal cells from excitotoxicity.
- Case Study : In rodent models of ischemia, administration of this compound resulted in reduced infarct size and improved neurological outcomes .
Pharmacokinetics and Toxicology
While detailed pharmacokinetic data for this compound remains limited, preliminary studies suggest:
- Absorption : Rapid absorption with potential for significant bioavailability.
- Metabolism : Metabolized primarily through oxidation processes leading to carboxylic acids or ketones.
- Toxicity : Initial assessments indicate low cytotoxicity at therapeutic doses; however, further studies are required to establish safety profiles comprehensively.
Q & A
Basic Research Questions
Q. What are the recommended synthetic routes for 1-(3-Ethynylphenyl)ethanone, and how do reaction conditions influence yield?
- Methodology : Friedel-Crafts acylation is a primary method, using acetyl chloride and a Lewis acid catalyst (e.g., AlCl₃) with 3-ethynylbenzene derivatives. Solvent choice (e.g., dichloromethane) and temperature control (0–25°C) are critical to avoid side reactions like over-acylation . Alternative routes include Sonogashira coupling between ethynyl precursors and acyl halides under palladium catalysis .
- Key Considerations : Monitor reaction progress via TLC or GC-MS to optimize stoichiometry and catalyst loading. Purification via column chromatography (silica gel, hexane/ethyl acetate) is recommended.
Q. How can researchers characterize this compound using spectroscopic and thermodynamic data?
- Methodology :
- NMR : Expect aromatic protons in the δ 7.2–8.1 ppm range (1H NMR) and a carbonyl signal at ~δ 2.6 ppm (13C NMR) .
- Mass Spectrometry : Base peak at m/z 144 (molecular ion [M⁺]) with fragmentation patterns reflecting ethynyl and acetyl groups .
- Boiling Point : Estimated at ~469–493 K based on analogs like 1-(3-methylphenyl)ethanone .
Q. What safety protocols are essential for handling this compound in laboratory settings?
- Guidelines :
- Use PPE (gloves, goggles, lab coat) to avoid skin/eye contact.
- Work under fume hoods to prevent inhalation of vapors/dust .
- Store in airtight containers away from oxidizing agents due to the ethynyl group’s reactivity .
- Emergency Measures : In case of exposure, rinse with water for 15 minutes and seek medical evaluation .
Q. How does the ethynyl substituent influence the compound’s reactivity in organic transformations?
- Mechanistic Insight : The ethynyl group enables click chemistry (e.g., Huisgen cycloaddition with azides) and Sonogashira coupling for functionalization. Steric effects from the acetyl group may slow reactions compared to non-acetylated analogs .
- Experimental Design : Use Cu(I) catalysts for regioselective alkyne-azide cycloaddition and monitor via IR (disappearance of ≡C-H stretch at ~3300 cm⁻¹) .
Advanced Research Questions
Q. How can computational modeling predict the biological activity of this compound derivatives?
- Methodology :
- Molecular Docking : Use AutoDock Vina or Schrödinger Suite to simulate binding to target proteins (e.g., kinases, enzymes). Optimize ligand conformers and score binding energies .
- ADMET Prediction : Employ SwissADME to assess Lipinski’s rule compliance (e.g., logP < 5, molecular weight < 500 Da) and toxicity endpoints .
- Case Study : Derivatives with electron-withdrawing groups show enhanced binding to CYP450 enzymes in silico .
Q. What strategies resolve contradictions in spectral data for structurally similar ethanones?
- Case Example : Conflicting NMR signals in 1-(2,3-dihydroxyphenyl)ethanone isomers can be resolved via 2D-COSY and HSQC to assign proton coupling and differentiate ortho/meta substitution .
- Validation : Cross-reference with X-ray crystallography (if crystalline) or computational NMR prediction tools (e.g., ACD/Labs) .
Q. How do reaction conditions impact regioselectivity in Friedel-Crafts acylation of ethynyl-substituted aromatics?
- Experimental Design :
- Catalyst Screening : Compare AlCl₃, FeCl₃, and ionic liquids for para/meta selectivity.
- Solvent Effects : Polar aprotic solvents (e.g., nitrobenzene) favor para-acylation, while non-polar solvents increase meta products .
- Data Analysis : Use HPLC to quantify isomer ratios and DFT calculations to model transition states .
Q. What are the challenges in scaling up synthesis while maintaining purity for pharmacological studies?
- Optimization :
- Continuous Flow Reactors : Enhance heat/mass transfer for reproducible yields .
- Crystallization : Use solvent mixtures (ethanol/water) to improve crystal purity. Monitor polymorphs via PXRD .
Q. How can structure-activity relationships (SAR) guide the design of this compound-based inhibitors?
- Methodology :
- Electron Density Mapping : Introduce substituents (e.g., -NO₂, -OH) at specific positions to modulate hydrogen bonding with target residues .
- Biological Assays : Test analogs against enzyme panels (e.g., kinases) and correlate IC₅₀ values with computed electrostatic potentials .
Q. What advanced techniques validate the environmental stability of this compound and its metabolites?
- Analytical Workflow :
- Photodegradation Studies : Expose to UV light (λ = 254 nm) and analyze degradation products via LC-QTOF-MS .
- Ecotoxicity : Use Daphnia magna assays to assess acute toxicity (LC₅₀) and QSAR models to predict bioaccumulation .
- Regulatory Compliance : Align with OECD guidelines for chemical safety assessments .
Properties
IUPAC Name |
1-(3-ethynylphenyl)ethanone | |
---|---|---|
Source | PubChem | |
URL | https://pubchem.ncbi.nlm.nih.gov | |
Description | Data deposited in or computed by PubChem | |
InChI |
InChI=1S/C10H8O/c1-3-9-5-4-6-10(7-9)8(2)11/h1,4-7H,2H3 | |
Source | PubChem | |
URL | https://pubchem.ncbi.nlm.nih.gov | |
Description | Data deposited in or computed by PubChem | |
InChI Key |
UVDLFLCDFUZLBZ-UHFFFAOYSA-N | |
Source | PubChem | |
URL | https://pubchem.ncbi.nlm.nih.gov | |
Description | Data deposited in or computed by PubChem | |
Canonical SMILES |
CC(=O)C1=CC=CC(=C1)C#C | |
Source | PubChem | |
URL | https://pubchem.ncbi.nlm.nih.gov | |
Description | Data deposited in or computed by PubChem | |
Molecular Formula |
C10H8O | |
Source | PubChem | |
URL | https://pubchem.ncbi.nlm.nih.gov | |
Description | Data deposited in or computed by PubChem | |
DSSTOX Substance ID |
DTXSID00569723 | |
Record name | 1-(3-Ethynylphenyl)ethan-1-one | |
Source | EPA DSSTox | |
URL | https://comptox.epa.gov/dashboard/DTXSID00569723 | |
Description | DSSTox provides a high quality public chemistry resource for supporting improved predictive toxicology. | |
Molecular Weight |
144.17 g/mol | |
Source | PubChem | |
URL | https://pubchem.ncbi.nlm.nih.gov | |
Description | Data deposited in or computed by PubChem | |
CAS No. |
139697-98-6 | |
Record name | 1-(3-Ethynylphenyl)ethan-1-one | |
Source | EPA DSSTox | |
URL | https://comptox.epa.gov/dashboard/DTXSID00569723 | |
Description | DSSTox provides a high quality public chemistry resource for supporting improved predictive toxicology. | |
Retrosynthesis Analysis
AI-Powered Synthesis Planning: Our tool employs the Template_relevance Pistachio, Template_relevance Bkms_metabolic, Template_relevance Pistachio_ringbreaker, Template_relevance Reaxys, Template_relevance Reaxys_biocatalysis model, leveraging a vast database of chemical reactions to predict feasible synthetic routes.
One-Step Synthesis Focus: Specifically designed for one-step synthesis, it provides concise and direct routes for your target compounds, streamlining the synthesis process.
Accurate Predictions: Utilizing the extensive PISTACHIO, BKMS_METABOLIC, PISTACHIO_RINGBREAKER, REAXYS, REAXYS_BIOCATALYSIS database, our tool offers high-accuracy predictions, reflecting the latest in chemical research and data.
Strategy Settings
Precursor scoring | Relevance Heuristic |
---|---|
Min. plausibility | 0.01 |
Model | Template_relevance |
Template Set | Pistachio/Bkms_metabolic/Pistachio_ringbreaker/Reaxys/Reaxys_biocatalysis |
Top-N result to add to graph | 6 |
Feasible Synthetic Routes
Disclaimer and Information on In-Vitro Research Products
Please be aware that all articles and product information presented on BenchChem are intended solely for informational purposes. The products available for purchase on BenchChem are specifically designed for in-vitro studies, which are conducted outside of living organisms. In-vitro studies, derived from the Latin term "in glass," involve experiments performed in controlled laboratory settings using cells or tissues. It is important to note that these products are not categorized as medicines or drugs, and they have not received approval from the FDA for the prevention, treatment, or cure of any medical condition, ailment, or disease. We must emphasize that any form of bodily introduction of these products into humans or animals is strictly prohibited by law. It is essential to adhere to these guidelines to ensure compliance with legal and ethical standards in research and experimentation.