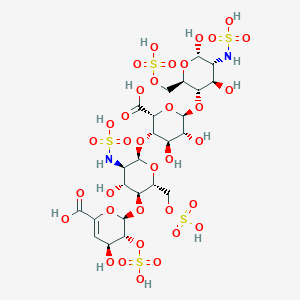
Heparin-derived tetrasaccharide
Overview
Description
Heparin-derived tetrasaccharides are low-molecular-weight oligosaccharides generated through enzymatic or chemical depolymerization of heparin, a sulfated glycosaminoglycan (GAG) . Structurally, these tetrasaccharides consist of four monosaccharide units: a ΔUA(2S) (unsaturated 2-O-sulfated uronic acid), a GlcNS6S (N-sulfated glucosamine-6-O-sulfate), an IdoA(2S) (2-O-sulfated iduronic acid), and another GlcNS6S residue . Key structural features include sulfation patterns (2-O, 6-O, and N-sulfation) and conformational flexibility of the iduronic acid (IdoA) residue, which adopts a distorted boat or chair conformation depending on the environment .
These tetrasaccharides are critical for studying heparin-protein interactions, such as binding to fibroblast growth factor-2 (FGF-2) and antithrombin III (ATIII), due to their well-defined sulfation motifs . Advanced NMR techniques (e.g., NOESY, TOCSY) and computational modeling (e.g., IRMA, molecular dynamics) have elucidated their solution conformations, revealing a right-handed helical structure with sulfate groups oriented equatorially .
Preparation Methods
Synthetic Routes and Reaction Conditions: Heparin-derived tetrasaccharides can be synthesized through a combination of chemical modification and enzymatic digestion. One common method involves the partial digestion of heparin using heparinase I, followed by purification through chromatography . The stereoselective synthesis of these tetrasaccharides often requires precise control over reaction conditions to ensure the correct stereochemistry and sulfation patterns .
Industrial Production Methods: Industrial production of heparin-derived tetrasaccharides typically involves the extraction of heparin from animal tissues, followed by controlled enzymatic digestion and purification. Advances in recombinant enzyme technology and metabolic engineering have also enabled the production of these compounds using microbial systems .
Chemical Reactions Analysis
Types of Reactions: Heparin-derived tetrasaccharides undergo various chemical reactions, including oxidation, reduction, and substitution. These reactions are essential for modifying the structure and function of the tetrasaccharides.
Common Reagents and Conditions: Common reagents used in these reactions include heparinases for enzymatic digestion, sodium periodate for oxidation, and reducing agents like sodium borohydride . Reaction conditions often involve controlled pH and temperature to ensure specificity and yield.
Major Products: The major products formed from these reactions include various sulfated and non-sulfated tetrasaccharide fragments, each with distinct biological activities .
Scientific Research Applications
Biological Interactions and Mechanisms
Heparin-derived tetrasaccharides are known to mediate critical biological interactions, particularly in the context of inflammation and immune response. Studies have demonstrated their ability to inhibit specific pathways in the complement system and chemokine receptor binding.
Complement System Inhibition:
- Heparin-derived tetrasaccharides have been shown to selectively inhibit the lectin pathway of the complement system. For instance, a study indicated that specific tetrasaccharides reduced the binding of MASP-2, a key protease in this pathway, by approximately 40% .
- The effectiveness of these tetrasaccharides varies based on their structural modifications. A fully hexasulfated tetrasaccharide exhibited stronger inhibition compared to its synthetic counterparts .
Chemokine Interaction:
- Tetrasaccharides derived from heparin can inhibit the binding of chemokines such as CCL5 to their receptors (e.g., CCR1). This inhibition is crucial for modulating inflammatory responses, with IC50 values indicating varying degrees of efficacy depending on the specific tetrasaccharide used .
Therapeutic Applications
The therapeutic potential of heparin-derived tetrasaccharides extends across several medical fields:
2.1. Cancer Therapy
- Heparin-derived tetrasaccharides have been explored for their ability to inhibit tumor growth and metastasis. Their interaction with growth factors and cytokines can modulate tumor microenvironments, making them promising candidates for cancer treatment .
2.2. Drug Delivery Systems
- Due to their biocompatibility and ability to bind various biomolecules, heparin-derived tetrasaccharides are being investigated as carriers for drug delivery systems. They can enhance the stability and bioavailability of therapeutic agents while reducing side effects .
2.3. Tissue Engineering
- In tissue engineering, heparin-derived tetrasaccharides contribute to scaffold design by promoting cell adhesion and growth factor retention. Their incorporation into hydrogels has shown potential in enhancing tissue regeneration processes .
Structural Characterization
Understanding the structure of heparin-derived tetrasaccharides is essential for elucidating their functional properties:
Tetrasaccharide Structure | Components | Biological Activity |
---|---|---|
1. ΔU2S(1→4)-α-D-GlcNS6S(1→4)-α-L-Idu2S(1→4)-α-D-GlcNS6S | Iduronic acid, Glucosamine | Moderate anticoagulant activity |
2. ΔU2S(1→4)-α-D-GlcNS6S(1→4)-β-D-GlcA(1→4)-α-D-GlcNS6S | Iduronic acid, Glucosamine | Low anticoagulant activity |
3. ΔU2S(1→4)-α-D-GlcNAc(1→4)-β-D-GlcA(1→4)-α-D-GlcNS6S | Iduronic acid, N-acetylglucosamine | Non-significant activity |
The structural diversity among heparin-derived tetrasaccharides correlates with their biological activities, highlighting the importance of specific glycosidic linkages and sulfation patterns in determining functionality .
Case Studies
Case Study 1: Inhibition of Inflammation
A study demonstrated that heparin-derived tetrasaccharides effectively reduced inflammation in an animal model by inhibiting CCL5 binding to CCR1 receptors. This suggests potential applications in treating inflammatory diseases .
Case Study 2: Drug Delivery Enhancement
Research has shown that incorporating heparin-derived tetrasaccharides into nanoparticle formulations significantly improved the delivery efficiency of anticancer drugs, enhancing therapeutic outcomes in preclinical models .
Mechanism of Action
The primary mechanism of action of heparin-derived tetrasaccharides involves their interaction with antithrombin III. This interaction enhances the inhibitory activity of antithrombin against serine proteases like thrombin and factor Xa, leading to anticoagulant effects . The binding of these tetrasaccharides induces conformational changes in antithrombin, increasing its affinity for target proteases .
Comparison with Similar Compounds
Structural and Conformational Comparisons
Key Findings :
- Sulfation and Binding: Heparin tetrasaccharides exhibit stronger binding to FGF-2 (Kd ~10 nM) compared to non-sulfated analogs (e.g., hyaluronic tetrasaccharides, Kd >1 µM) due to electrostatic interactions with sulfates .
- IdoA Flexibility : Unlike GlcA in HS tetrasaccharides, IdoA(2S) in heparin tetrasaccharides adopts a dynamic equilibrium between $ ^1C4 $ and $ ^2S0 $ conformers, enabling adaptive binding to proteins .
Key Findings :
- 3-O-Sulfation Specificity: Heparin tetrasaccharides with a 3-O-sulfated GlcNS residue (e.g., ATIII-binding sequences) show 100-fold higher anticoagulant activity than non-3-O-sulfated variants .
- Glycomimetics : HS glycomimetics with triazole spacers exhibit altered end-to-end distances (2.1 nm vs. 1.5 nm in native HS), reducing FGF-2 binding efficacy .
Key Findings :
- Electron Detachment Dissociation (EDD): Enables precise identification of sulfation sites in heparin tetrasaccharides, unlike IRMPD, which fragments non-specifically .
- Synthetic Routes : Heparin tetrasaccharides are typically isolated from natural sources, while analogs like GM2 are synthesized via α-selective sialylation .
Research Findings and Controversies
- Contradictions in Sulfate Orientation : While NMR data suggest heparin tetrasaccharides predominantly adopt a gt conformation for 6-O-sulfates, X-ray crystallography of FGF-2-bound structures shows gg conformations, indicating context-dependent flexibility .
- Conformational Dynamics : Molecular dynamics simulations reveal that heparin tetrasaccharides in solution sample multiple rotamers, with energy barriers <2 kcal/mol for IdoA(2S) ring flipping .
Q & A
Basic Research Questions
Q. How is the three-dimensional structure of heparin-derived tetrasaccharide determined experimentally?
Nuclear magnetic resonance (NMR) spectroscopy is the primary method for resolving its solution conformation. Key parameters include:
- Coupling constants (³JH-H): Used to identify glycosidic linkage conformations (e.g., 3JH1-H2 = 2.51 Hz and 3JH4-H5 = 2.23 Hz in free tetrasaccharide, indicating equilibrium between 1C4 and 2S0 conformers .
- Transferred NOEs : Detect ligand-protein interactions (e.g., stronger NOEs at GlcN residues during antithrombin binding) .
- Chemical shift perturbations : Highlight residues involved in protein binding (e.g., GlcN 6-SO3 and GlcN 3,6-SO3 units show the largest shifts) . Molecular dynamics (MD) simulations complement NMR data to model conformational dynamics .
Q. What in vitro assays are used to quantify this compound-protein interactions?
- Isothermal titration calorimetry (ITC) : Measures binding thermodynamics (ΔH, Kd, stoichiometry). For example, this compound binds secretory leukocyte protease inhibitor (SLPI) with Kd = 197 μM and ΔH = 22.7 kcal/mol .
- Surface plasmon resonance (SPR) : Provides real-time kinetics (kon/koff) for high-affinity interactions (e.g., antithrombin binding) .
- Competitive ELISA : Quantifies inhibition of protein-GAG interactions (e.g., factor H binding in age-related macular degeneration studies) .
Q. What structural features of this compound are critical for biological activity?
Key features include:
- Sulfation pattern : 3-O-sulfation on GlcN residues is essential for high-affinity antithrombin binding .
- Iduronic acid (IdoA) conformation : The 2S0 → 1C4 shift upon protein binding enhances complementarity .
- Carboxylic acid pKa : Determined via NMR titration (pKa ≈ 3.2–3.5 for IdoA residues), influencing charge distribution .
Q. Which analytical techniques are used for sequencing and purity assessment of heparin-derived tetrasaccharides?
- SAX-ESI-MS/MS : Separates oligosaccharides by charge and mass (e.g., distinguishes glycol-split heparins) .
- Capillary electrophoresis (CE) : Resolves sulfation isomers with high resolution .
- HILIC-MS : Profiles tetrasaccharides in complex matrices (e.g., human milk metabolomics) .
Advanced Research Questions
Q. How do conformational dynamics of this compound influence protein binding?
NMR and MD simulations reveal a two-step binding mechanism:
- Step 1 : Low-affinity recognition induces conformational changes (e.g., GlcN 6-SO3-GlcA linkage shifts to fit antithrombin’s binding site) .
- Step 2 : High-affinity binding stabilizes the 1C4 chair form of IdoA, enhancing electrostatic complementarity . Thermodynamic studies (ITC) show entropy-driven binding for highly sulfated variants .
Q. How can researchers resolve contradictions in binding affinity data across studies?
- Multi-method validation : Combine ITC (thermodynamics), SPR (kinetics), and NMR (structural data) to cross-verify results .
- Control for sulfation heterogeneity : Use ultra-pure tetrasaccharides (e.g., synthetic standards) to minimize batch variability .
- Context-dependent analysis : Account for pH, ionic strength, and protein isoforms (e.g., factor H SCR6-8 vs. SCR7 binding) .
Q. What advanced strategies are used to analyze sulfation isomers of heparin-derived tetrasaccharides?
- Ion mobility spectrometry (IMS)-MS : Separates isomers based on collision cross-section differences .
- Enzymatic sequencing : Heparinases I/II/III generate diagnostic fragments (e.g., ΔUA-GlcN vs. ΔUA-IdoA linkages) .
- Tandem MS<sup>n</sup> : Fragmentation patterns (e.g., Y/Z ions) differentiate 3-O-sulfated vs. 6-O-sulfated GlcN residues .
Q. How do non-sulfated residues in heparin-derived tetrasaccharides contribute to biological function?
- Glycol-split residues : Engineered non-sulfated GlcA residues in glycol-split heparins enhance anti-angiogenic activity by altering chain flexibility .
- Carboxyl group interactions : Protonation states (pKa ~3.5) mediate hydrogen bonding with lysine-rich protein domains .
Q. What methodologies determine the pKa values of carboxylic acid groups in heparin-derived tetrasaccharides?
- NMR pH titration : Monitors chemical shifts of H4 (ΔUA) and H5 (IdoA) protons. For example, pKa = 3.4 ± 0.1 for IdoA in hexasaccharides .
- Density functional theory (DFT) : Predicts pKa shifts caused by sulfation patterns .
Q. How can researchers design this compound mutants for structure-activity studies?
- Chemoenzymatic synthesis : Uses recombinant sulfotransferases (e.g., 3-OST-1) to introduce site-specific sulfation .
- Alanine scanning mutagenesis : Replaces key sulfate groups (e.g., 6-O-sulfate on GlcN) to assess binding energy contributions .
- SPR-guided design : Iteratively optimizes mutants based on binding kinetics (koff < 10<sup>−3</sup> s<sup>−1</sup> for high-affinity variants) .
Properties
IUPAC Name |
(2R,3R,4S)-2-[(2R,3S,4R,5R,6R)-6-[(2R,3S,4R,5R,6R)-2-carboxy-6-[(2R,3S,4R,5R,6S)-4,6-dihydroxy-5-(sulfoamino)-2-(sulfooxymethyl)oxan-3-yl]oxy-4,5-dihydroxyoxan-3-yl]oxy-4-hydroxy-5-(sulfoamino)-2-(sulfooxymethyl)oxan-3-yl]oxy-4-hydroxy-3-sulfooxy-3,4-dihydro-2H-pyran-6-carboxylic acid | |
---|---|---|
Source | PubChem | |
URL | https://pubchem.ncbi.nlm.nih.gov | |
Description | Data deposited in or computed by PubChem | |
InChI |
InChI=1S/C24H38N2O35S5/c27-4-1-5(19(32)33)55-24(14(4)61-66(49,50)51)58-16-7(3-53-65(46,47)48)56-22(9(11(16)29)26-63(40,41)42)59-17-12(30)13(31)23(60-18(17)20(34)35)57-15-6(2-52-64(43,44)45)54-21(36)8(10(15)28)25-62(37,38)39/h1,4,6-18,21-31,36H,2-3H2,(H,32,33)(H,34,35)(H,37,38,39)(H,40,41,42)(H,43,44,45)(H,46,47,48)(H,49,50,51)/t4-,6+,7+,8+,9+,10+,11+,12+,13+,14+,15+,16+,17-,18+,21-,22+,23+,24-/m0/s1 | |
Source | PubChem | |
URL | https://pubchem.ncbi.nlm.nih.gov | |
Description | Data deposited in or computed by PubChem | |
InChI Key |
QTSOBXZFMHJWGX-UQSIALEXSA-N | |
Source | PubChem | |
URL | https://pubchem.ncbi.nlm.nih.gov | |
Description | Data deposited in or computed by PubChem | |
Canonical SMILES |
C1=C(OC(C(C1O)OS(=O)(=O)O)OC2C(OC(C(C2O)NS(=O)(=O)O)OC3C(C(C(OC3C(=O)O)OC4C(OC(C(C4O)NS(=O)(=O)O)O)COS(=O)(=O)O)O)O)COS(=O)(=O)O)C(=O)O | |
Source | PubChem | |
URL | https://pubchem.ncbi.nlm.nih.gov | |
Description | Data deposited in or computed by PubChem | |
Isomeric SMILES |
C1=C(O[C@H]([C@@H]([C@H]1O)OS(=O)(=O)O)O[C@@H]2[C@H](O[C@@H]([C@@H]([C@H]2O)NS(=O)(=O)O)O[C@H]3[C@@H]([C@H]([C@@H](O[C@H]3C(=O)O)O[C@@H]4[C@H](O[C@@H]([C@@H]([C@H]4O)NS(=O)(=O)O)O)COS(=O)(=O)O)O)O)COS(=O)(=O)O)C(=O)O | |
Source | PubChem | |
URL | https://pubchem.ncbi.nlm.nih.gov | |
Description | Data deposited in or computed by PubChem | |
Molecular Formula |
C24H38N2O35S5 | |
Source | PubChem | |
URL | https://pubchem.ncbi.nlm.nih.gov | |
Description | Data deposited in or computed by PubChem | |
Molecular Weight |
1074.9 g/mol | |
Source | PubChem | |
URL | https://pubchem.ncbi.nlm.nih.gov | |
Description | Data deposited in or computed by PubChem | |
Retrosynthesis Analysis
AI-Powered Synthesis Planning: Our tool employs the Template_relevance Pistachio, Template_relevance Bkms_metabolic, Template_relevance Pistachio_ringbreaker, Template_relevance Reaxys, Template_relevance Reaxys_biocatalysis model, leveraging a vast database of chemical reactions to predict feasible synthetic routes.
One-Step Synthesis Focus: Specifically designed for one-step synthesis, it provides concise and direct routes for your target compounds, streamlining the synthesis process.
Accurate Predictions: Utilizing the extensive PISTACHIO, BKMS_METABOLIC, PISTACHIO_RINGBREAKER, REAXYS, REAXYS_BIOCATALYSIS database, our tool offers high-accuracy predictions, reflecting the latest in chemical research and data.
Strategy Settings
Precursor scoring | Relevance Heuristic |
---|---|
Min. plausibility | 0.01 |
Model | Template_relevance |
Template Set | Pistachio/Bkms_metabolic/Pistachio_ringbreaker/Reaxys/Reaxys_biocatalysis |
Top-N result to add to graph | 6 |
Feasible Synthetic Routes
Disclaimer and Information on In-Vitro Research Products
Please be aware that all articles and product information presented on BenchChem are intended solely for informational purposes. The products available for purchase on BenchChem are specifically designed for in-vitro studies, which are conducted outside of living organisms. In-vitro studies, derived from the Latin term "in glass," involve experiments performed in controlled laboratory settings using cells or tissues. It is important to note that these products are not categorized as medicines or drugs, and they have not received approval from the FDA for the prevention, treatment, or cure of any medical condition, ailment, or disease. We must emphasize that any form of bodily introduction of these products into humans or animals is strictly prohibited by law. It is essential to adhere to these guidelines to ensure compliance with legal and ethical standards in research and experimentation.