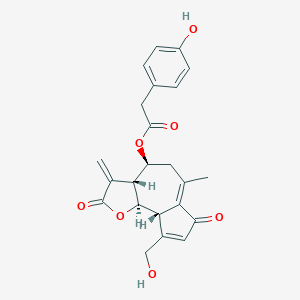
Lactupicrin
Overview
Description
Mechanism of Action
Target of Action
Lactupicrin, also known as Lactucopicrin, is a sesquiterpene lactone . It primarily targets the central nervous system , where it exhibits sedative and analgesic effects . It has also been shown to act as an acetylcholinesterase inhibitor , which plays a crucial role in nerve signal transmission.
Mode of Action
This compound interacts with its targets by acting on the central nervous system, providing sedative and analgesic effects . As an acetylcholinesterase inhibitor, it prevents the breakdown of acetylcholine, a neurotransmitter essential for communication between nerve cells . This results in an increase in the concentration of acetylcholine, enhancing nerve signal transmission.
Biochemical Pathways
This compound affects several biochemical pathways. It activates autophagy in cells, a process that removes unnecessary or dysfunctional components . It also influences cell signaling pathways, specifically decreasing the phosphorylation of proliferative signals pAKT and pERK . These changes can lead to various downstream effects, including cell cycle arrest and apoptosis .
Result of Action
The action of this compound leads to several molecular and cellular effects. It induces cell cycle arrest in the G2/M phase, confirmed by a decrease of CDK2 protein and an increase of p53 and p21 . It also stimulates apoptosis, as evidenced by a reduction of procaspase 6 and an increase of the cleaved/full-length PARP ratio . These effects contribute to its potential as a therapeutic agent.
Biochemical Analysis
Biochemical Properties
Lactupicrin plays a significant role in biochemical reactions, particularly in its interaction with enzymes and proteins. One of the key interactions is with acetylcholinesterase, an enzyme responsible for breaking down the neurotransmitter acetylcholine. This compound inhibits acetylcholinesterase, leading to increased levels of acetylcholine in the synaptic cleft, which can enhance cholinergic transmission . Additionally, this compound has been shown to interact with various proteins involved in oxidative stress and cell cycle regulation, such as p53 and p21 .
Cellular Effects
This compound exerts various effects on different cell types and cellular processes. In glioblastoma cells, this compound has been shown to induce autophagy, cell cycle arrest, and oxidative stress . It decreases the phosphorylation of proliferative signals such as pAKT and pERK, leading to reduced cell proliferation. This compound also stimulates apoptosis by increasing the cleaved/full-length PARP ratio and reducing procaspase 6 levels . These effects highlight its potential as an anticancer agent.
Molecular Mechanism
The molecular mechanism of this compound involves several pathways. It binds to acetylcholinesterase, inhibiting its activity and increasing acetylcholine levels . This compound also induces oxidative stress by generating reactive oxygen species (ROS), which can damage cellular components and lead to cell death . Furthermore, it activates autophagy and induces cell cycle arrest by modulating the expression of key regulatory proteins such as p53, p21, and CDK2 . These combined actions contribute to its cytotoxic effects on cancer cells.
Temporal Effects in Laboratory Settings
In laboratory settings, the effects of this compound have been observed to change over time. Studies have shown that daily administration of this compound leads to a dose- and time-dependent reduction in glioblastoma cell growth and viability . The compound’s stability and degradation over time can influence its long-term effects on cellular function. For instance, prolonged exposure to this compound can enhance its cytotoxic effects through sustained oxidative stress and autophagy activation .
Dosage Effects in Animal Models
The effects of this compound vary with different dosages in animal models. At lower doses, this compound has been shown to have neuroprotective effects by inhibiting acetylcholinesterase and enhancing cholinergic transmission . At higher doses, it can induce toxic effects, including oxidative stress and apoptosis . These dose-dependent effects highlight the importance of determining the optimal therapeutic dosage to maximize benefits while minimizing adverse effects.
Metabolic Pathways
This compound is involved in several metabolic pathways, including those related to oxidative stress and cell cycle regulation. It interacts with enzymes such as acetylcholinesterase and proteins involved in the regulation of oxidative stress, such as p53 and p21 . These interactions can influence metabolic flux and metabolite levels, contributing to its overall biochemical effects.
Transport and Distribution
This compound is transported and distributed within cells and tissues through various mechanisms. It can interact with transporters and binding proteins that facilitate its movement across cell membranes . Once inside the cell, this compound can accumulate in specific compartments, influencing its localization and activity. For example, its interaction with acetylcholinesterase occurs primarily in the synaptic cleft, where it can modulate cholinergic transmission .
Subcellular Localization
The subcellular localization of this compound is crucial for its activity and function. It can be directed to specific compartments or organelles through targeting signals and post-translational modifications . For instance, this compound’s interaction with acetylcholinesterase occurs in the synaptic cleft, while its effects on oxidative stress and autophagy are mediated through its localization in the cytoplasm and mitochondria . These localization patterns are essential for understanding its mechanism of action and therapeutic potential.
Preparation Methods
Synthetic Routes and Reaction Conditions
Lactucopicrin can be isolated from plant sources using medium-pressure preparative liquid chromatography (MPPLC). The process involves extracting the methanolic extract of the whole plant with petroleum ether and ethyl acetate to obtain the ethyl acetate extract. The crude products, including lactucopicrin, are then separated using MPPLC and thin-layer chromatography (TLC) tracking .
Industrial Production Methods
Industrial production of lactucopicrin primarily relies on the extraction from plant sources such as wild lettuce and chicory. The process involves harvesting the plants, extracting the latex, and purifying the compound using chromatographic techniques .
Chemical Reactions Analysis
Types of Reactions
Lactucopicrin undergoes various chemical reactions, including:
Oxidation: Lactucopicrin can be oxidized to form different derivatives.
Reduction: Reduction reactions can modify the lactone ring structure.
Substitution: Substitution reactions can occur at the hydroxyl and acetoxy groups.
Common Reagents and Conditions
Common reagents used in these reactions include oxidizing agents like potassium permanganate, reducing agents like sodium borohydride, and various acids and bases for substitution reactions .
Major Products Formed
The major products formed from these reactions include oxidized derivatives, reduced lactone rings, and substituted lactucopicrin derivatives .
Scientific Research Applications
Lactucopicrin has a wide range of scientific research applications:
Chemistry: Used as a model compound for studying sesquiterpene lactones and their chemical properties.
Biology: Investigated for its role in plant defense mechanisms and its interactions with herbivores.
Medicine: Studied for its sedative, analgesic, antimalarial, and acetylcholinesterase inhibitory activities
Industry: Utilized in the production of natural pesticides and pharmaceuticals.
Comparison with Similar Compounds
Similar Compounds
Dihydrolactucin: A reduced form of lactucin with similar biological activities.
Uniqueness of Lactucopicrin
Lactucopicrin is unique due to its combination of sedative, analgesic, antimalarial, and acetylcholinesterase inhibitory activities. Its diverse range of biological effects makes it a valuable compound for various scientific and industrial applications .
Properties
IUPAC Name |
[9-(hydroxymethyl)-6-methyl-3-methylidene-2,7-dioxo-4,5,9a,9b-tetrahydro-3aH-azuleno[4,5-b]furan-4-yl] 2-(4-hydroxyphenyl)acetate | |
---|---|---|
Details | Computed by Lexichem TK 2.7.0 (PubChem release 2021.05.07) | |
Source | PubChem | |
URL | https://pubchem.ncbi.nlm.nih.gov | |
Description | Data deposited in or computed by PubChem | |
InChI |
InChI=1S/C23H22O7/c1-11-7-17(29-18(27)8-13-3-5-15(25)6-4-13)20-12(2)23(28)30-22(20)21-14(10-24)9-16(26)19(11)21/h3-6,9,17,20-22,24-25H,2,7-8,10H2,1H3 | |
Details | Computed by InChI 1.0.6 (PubChem release 2021.05.07) | |
Source | PubChem | |
URL | https://pubchem.ncbi.nlm.nih.gov | |
Description | Data deposited in or computed by PubChem | |
InChI Key |
UMVSOHBRAQTGQI-UHFFFAOYSA-N | |
Details | Computed by InChI 1.0.6 (PubChem release 2021.05.07) | |
Source | PubChem | |
URL | https://pubchem.ncbi.nlm.nih.gov | |
Description | Data deposited in or computed by PubChem | |
Canonical SMILES |
CC1=C2C(C3C(C(C1)OC(=O)CC4=CC=C(C=C4)O)C(=C)C(=O)O3)C(=CC2=O)CO | |
Details | Computed by OEChem 2.3.0 (PubChem release 2021.05.07) | |
Source | PubChem | |
URL | https://pubchem.ncbi.nlm.nih.gov | |
Description | Data deposited in or computed by PubChem | |
Molecular Formula |
C23H22O7 | |
Details | Computed by PubChem 2.1 (PubChem release 2021.05.07) | |
Source | PubChem | |
URL | https://pubchem.ncbi.nlm.nih.gov | |
Description | Data deposited in or computed by PubChem | |
Molecular Weight |
410.4 g/mol | |
Details | Computed by PubChem 2.1 (PubChem release 2021.05.07) | |
Source | PubChem | |
URL | https://pubchem.ncbi.nlm.nih.gov | |
Description | Data deposited in or computed by PubChem | |
Physical Description |
Solid | |
Record name | Lactupicrin | |
Source | Human Metabolome Database (HMDB) | |
URL | http://www.hmdb.ca/metabolites/HMDB0035828 | |
Description | The Human Metabolome Database (HMDB) is a freely available electronic database containing detailed information about small molecule metabolites found in the human body. | |
Explanation | HMDB is offered to the public as a freely available resource. Use and re-distribution of the data, in whole or in part, for commercial purposes requires explicit permission of the authors and explicit acknowledgment of the source material (HMDB) and the original publication (see the HMDB citing page). We ask that users who download significant portions of the database cite the HMDB paper in any resulting publications. | |
CAS No. |
65725-11-3 | |
Record name | Lactupicrin | |
Source | Human Metabolome Database (HMDB) | |
URL | http://www.hmdb.ca/metabolites/HMDB0035828 | |
Description | The Human Metabolome Database (HMDB) is a freely available electronic database containing detailed information about small molecule metabolites found in the human body. | |
Explanation | HMDB is offered to the public as a freely available resource. Use and re-distribution of the data, in whole or in part, for commercial purposes requires explicit permission of the authors and explicit acknowledgment of the source material (HMDB) and the original publication (see the HMDB citing page). We ask that users who download significant portions of the database cite the HMDB paper in any resulting publications. | |
Melting Point |
132 - 178 °C | |
Record name | Lactupicrin | |
Source | Human Metabolome Database (HMDB) | |
URL | http://www.hmdb.ca/metabolites/HMDB0035828 | |
Description | The Human Metabolome Database (HMDB) is a freely available electronic database containing detailed information about small molecule metabolites found in the human body. | |
Explanation | HMDB is offered to the public as a freely available resource. Use and re-distribution of the data, in whole or in part, for commercial purposes requires explicit permission of the authors and explicit acknowledgment of the source material (HMDB) and the original publication (see the HMDB citing page). We ask that users who download significant portions of the database cite the HMDB paper in any resulting publications. | |
Retrosynthesis Analysis
AI-Powered Synthesis Planning: Our tool employs the Template_relevance Pistachio, Template_relevance Bkms_metabolic, Template_relevance Pistachio_ringbreaker, Template_relevance Reaxys, Template_relevance Reaxys_biocatalysis model, leveraging a vast database of chemical reactions to predict feasible synthetic routes.
One-Step Synthesis Focus: Specifically designed for one-step synthesis, it provides concise and direct routes for your target compounds, streamlining the synthesis process.
Accurate Predictions: Utilizing the extensive PISTACHIO, BKMS_METABOLIC, PISTACHIO_RINGBREAKER, REAXYS, REAXYS_BIOCATALYSIS database, our tool offers high-accuracy predictions, reflecting the latest in chemical research and data.
Strategy Settings
Precursor scoring | Relevance Heuristic |
---|---|
Min. plausibility | 0.01 |
Model | Template_relevance |
Template Set | Pistachio/Bkms_metabolic/Pistachio_ringbreaker/Reaxys/Reaxys_biocatalysis |
Top-N result to add to graph | 6 |
Feasible Synthetic Routes
Q1: How does Lactucopicrin exert its anti-inflammatory effects?
A1: Lactucopicrin inhibits the activation of nuclear factor-κB (NF-κB) in inflamed macrophages, which in turn reduces the expression of pro-inflammatory cytokines like IL-1β, IL-6, and tumor necrosis factor alpha []. This effect is attributed to Lactucopicrin's ability to inhibit cytoplasmic dynein-mediated transportation of the p65 subunit, a crucial step for NF-κB activation [].
Q2: What is Lactucopicrin's role in regulating lipid metabolism?
A2: Lactucopicrin promotes fatty acid β-oxidation by activating the adenosine monophosphate-activated protein kinase (AMPK) pathway []. This activation leads to the upregulation of crucial factors involved in fatty acid β-oxidation, including PPARα, PPARγ, CPT1A, and HADHA []. Consequently, Lactucopicrin attenuates lipid accumulation and improves mitochondrial function in hepatocytes [].
Q3: How does Lactucopicrin impact macrophage foam cell formation?
A3: Lactucopicrin limits macrophage foam cell formation by reducing the amount of lectin-like oxidized low-density lipoprotein receptor-1 (LOX-1) in lipid rafts []. This reduction in LOX-1 distribution disrupts the uptake of oxidized low-density lipoprotein (oxLDL), a key contributor to foam cell formation, without affecting cholesterol efflux [].
Q4: What is the molecular formula and weight of Lactucopicrin?
A4: Lactucopicrin has the molecular formula C23H22O7 and a molecular weight of 410.41 g/mol.
Q5: What spectroscopic techniques are commonly used to characterize Lactucopicrin?
A5: Various techniques are used, including high-performance liquid chromatography (HPLC) for separation and quantification [, , , , , ], proton nuclear magnetic resonance (NMR) for structural elucidation [], and liquid chromatography-mass spectrometry (LC-MS) for identification and quantification [, ].
Q6: How does the storage of chicory roots affect Lactucopicrin content?
A6: Storage conditions and processing methods, such as drying and milling, can impact the Lactucopicrin content in chicory roots, ultimately influencing the composition of chicory extracts [].
Q7: How do different washing procedures impact Lactucopicrin levels in fresh-cut chicory?
A7: Washing fresh-cut chicory, particularly with warm water, can significantly reduce sesquiterpene lactone (SL) content, including Lactucopicrin, and bitterness []. This suggests that specific washing protocols can be employed to modulate bitterness and preserve desirable sensory attributes.
Q8: Can Lactucopicrin be chemically modified for potential applications?
A8: Yes, Lactucopicrin can undergo regioselective O-acylation at its primary alcohol group using enzymes like lipase B from Candida antarctica (CAL-B) []. This biocatalytic approach allows for the introduction of various ester chains, expanding the potential applications of Lactucopicrin derivatives in pharmaceuticals and biocontrol agents [].
Q9: Have molecular docking studies been conducted with Lactucopicrin?
A9: Molecular docking simulations have been employed to investigate the interaction of Lactucopicrin with various targets. These include: * α-Glucosidase: Studies revealed that Lactucopicrin exhibits a strong binding affinity for specific residues within the active cavity of α-glucosidase, suggesting a competitive inhibition mechanism []. * Anti-apoptotic proteins: Docking simulations demonstrated that Lactucopicrin could potentially bind to the active sites of anti-apoptotic proteins, forming stable complexes, which might contribute to its anticancer activity []. * SARS-CoV-2 main protease (MPro): Studies predicted strong binding of Lactucopicrin 15-oxalate with MPro, suggesting its potential as a multi-target-directed ligand against SARS-CoV-2 infection [].
Q10: How does the structure of Lactucopicrin relate to its bitterness?
A10: Lactucopicrin possesses a guaianolide structure, a common feature among bitter compounds found in chicory. Sensory analysis coupled with chemical characterization indicates a strong correlation between the increasing levels of Lactucopicrin and perceived bitterness in lettuce and chicory [, , ].
Q11: What is the impact of structural modifications on Lactucopicrin's activity?
A11: Inactivating the lactucin synthase enzyme, which converts 8-deoxylactucin to lactucin, leads to an accumulation of 8-deoxylactucin and its derivatives, including 11β,13-dihydro-8-deoxylactucin, in chicory taproots []. Interestingly, a supercritical fluid extract fraction enriched in these compounds demonstrated anti-inflammatory activity in an inflamed intestinal mucosa model []. This highlights the potential for modifying the Lactucopicrin biosynthetic pathway to enhance the production of specific derivatives with desired bioactivities.
Q12: How stable are Lactucopicrin and its derivatives?
A12: Lactucopicrin exists naturally in free and conjugated forms, such as oxalates and sulfates []. The oxalyl conjugates are generally unstable and readily hydrolyze back to the parent sesquiterpene lactone []. In contrast, the sulfate conjugates demonstrate greater stability [].
Q13: What is known about the bioavailability of Lactucopicrin from dietary sources?
A13: Studies on healthy volunteers consuming chicory juice revealed that Lactucopicrin exhibits low oral bioavailability []. A significant portion of ingested Lactucopicrin undergoes gut microbial metabolism and phase II metabolism, resulting in the detection of various metabolites, including glucuronide and sulfate conjugates, in urine and fecal samples [].
Q14: What cellular models have been used to investigate Lactucopicrin's effects?
A14: Several cell lines have been used to study Lactucopicrin's biological activity: * Macrophages: Mouse bone marrow-derived macrophages treated with lipopolysaccharide (LPS) are used to investigate Lactucopicrin's anti-inflammatory properties in the context of atherosclerosis []. * HepG2: This human hepatoblastoma cell line, treated with free fatty acids, serves as an in vitro model of non-alcoholic fatty liver disease (NAFLD) to elucidate Lactucopicrin's role in lipid metabolism []. * Saos-2: This human osteosarcoma cell line is used to evaluate Lactucopicrin's anticancer effects, specifically its impact on cell proliferation, migration, invasion, and apoptosis []. * SKMEL-5: This human skin cancer cell line is employed to explore Lactucopicrin's anticancer properties, particularly its effects on cell proliferation, apoptosis, cell cycle progression, and the m-TOR/PI3K/AKT signaling pathway []. * U87Mg: This glioblastoma cell line is used to investigate Lactucopicrin's anticancer effects, including its impact on autophagy, cell cycle arrest, oxidative stress, and synergy with temozolomide (TMZ) [].
Q15: Have there been any animal studies on Lactucopicrin?
A15: Yes, several animal models have been utilized to evaluate Lactucopicrin's therapeutic potential: * Atherosclerosis: Apolipoprotein E-deficient mice fed a high-fat diet are used to study Lactucopicrin's impact on atherogenesis. Results indicate a reduction in plaque area, macrophage accumulation, and inflammatory markers, suggesting a protective role against atherosclerosis []. * Sleep Enhancement: Rodent models, including pentobarbital-induced sleep and caffeine-induced insomnia models, have been employed to demonstrate Lactucopicrin's sleep-enhancing properties [, ]. These studies highlight the ability of Lactucopicrin to increase sleep duration, potentially through its interaction with GABAergic mechanisms.
Q16: How is Lactucopicrin typically quantified in plant material?
A16: High-performance liquid chromatography (HPLC) is commonly employed for the separation and quantification of Lactucopicrin in plant extracts [, , , , , ]. This technique allows for the simultaneous determination of Lactucopicrin alongside other related sesquiterpene lactones, providing a comprehensive profile of these compounds in various plant species and tissues.
Q17: How does the bitterness of chicory root extract compare to that of quinine?
A17: Sensory analysis comparing the bitterness of beverages prepared with either quinine or chicory root extract revealed distinct sensory profiles []. Notably, while the bitterness of quinine-containing beverages decreased significantly upon storage in daylight, the bitterness of beverages with chicory root extract remained stable []. This observation highlights the potential of chicory root extract as a natural alternative to quinine in certain food applications.
Q18: What is the historical context of using chicory for its bitter compounds?
A18: Chicory, specifically its roots, has been cultivated for centuries, initially as a coffee substitute due to its bitter taste profile []. The bitter compounds responsible for this characteristic taste were later identified as sesquiterpene lactones, including Lactucopicrin.
Q19: Can Lactucopicrin be used in combination with other plant extracts for synergistic effects?
A19: Combining romaine lettuce extract (RE), rich in Lactucopicrin, with skullcap root extract (SE) demonstrated synergistic effects on sleep enhancement in rodent models []. This combination significantly increased total sleep time and enhanced non-rapid eye movement (NREM) sleep, suggesting a potential application for managing sleep disorders [].
Disclaimer and Information on In-Vitro Research Products
Please be aware that all articles and product information presented on BenchChem are intended solely for informational purposes. The products available for purchase on BenchChem are specifically designed for in-vitro studies, which are conducted outside of living organisms. In-vitro studies, derived from the Latin term "in glass," involve experiments performed in controlled laboratory settings using cells or tissues. It is important to note that these products are not categorized as medicines or drugs, and they have not received approval from the FDA for the prevention, treatment, or cure of any medical condition, ailment, or disease. We must emphasize that any form of bodily introduction of these products into humans or animals is strictly prohibited by law. It is essential to adhere to these guidelines to ensure compliance with legal and ethical standards in research and experimentation.