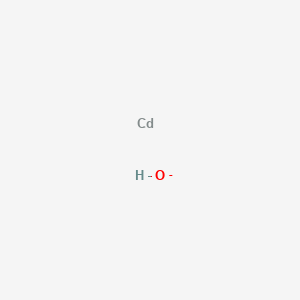
Cadmium;hydroxide
- Click on QUICK INQUIRY to receive a quote from our team of experts.
- With the quality product at a COMPETITIVE price, you can focus more on your research.
Overview
Description
Cadmium hydroxide (Cd(OH)₂) is a white crystalline solid with a hexagonal brucite-like structure. It is a weak base and a precursor to cadmium oxide (CdO), a semiconductor material widely used in solar cells, sensors, and transparent electrodes . Cd(OH)₂ is synthesized via hydrothermal methods, chemical precipitation, or laser ablation techniques . It exhibits n-type semiconducting properties and is notable for its role in producing cadmium chalcogenides (e.g., CdSe, CdS) and CdO through thermal decomposition .
Key properties of Cd(OH)₂ include:
Preparation Methods
Cadmium hydroxide can be synthesized through several methods:
Precipitation Method: This involves the addition of an alkaline solution, such as sodium hydroxide or potassium hydroxide, to a solution containing cadmium salts like cadmium nitrate. The reaction is as follows[ \text{Cd(NO}_3\text{)}_2 + 2\text{NaOH} \rightarrow \text{Cd(OH)}_2 + 2\text{NaNO}_3 ]
Hydrothermal and Solvothermal Methods: These methods involve the use of high temperature and pressure to synthesize cadmium hydroxide nanomaterials.
Microwave-Assisted Methods: This method uses microwave radiation to accelerate the reaction, resulting in the formation of cadmium hydroxide nanostructures.
Chemical Reactions Analysis
Thermal Decomposition
Cd(OH)₂ decomposes upon heating, forming cadmium oxide (CdO):
Cd(OH)2ΔCdO+H2O(130–300°C)[1][8]
Dehydration initiates at 130°C and completes at 300°C, with the oxide retaining Cd²⁺ in a crystalline lattice.
Reactions with Acids
Cadmium hydroxide reacts with mineral acids to form corresponding cadmium salts:
With HCl :
Cd(OH)2+2HCl→CdCl2+2H2O[1][4]
With H₂SO₄ :
Cd(OH)2+H2SO4→CdSO4+2H2O[1][4]
These reactions proceed rapidly in dilute acids due to the basic nature of Cd(OH)₂.
With Ammonia
Cd(OH)₂ dissolves in excess aqueous ammonia, forming the tetraammine complex:
Cd(OH)2+4NH3→[Cd(NH3)4]2++2OH−[1][3]
This complex is stable in alkaline conditions and is used in analytical separations.
With Hydroxide Ions
In strongly basic solutions (high [OH⁻]), Cd(OH)₂ forms hydroxo complexes:
Cd(OH)2+2OH−→[Cd(OH)4]2−[5][6]
Solubility increases with hydroxide concentration, as shown in Table 1 .
Table 1: Solubility of Cd(OH)₂ in NaOH Solutions (25°C)
[NaOH] (M) | Solubility (×10⁻⁵ mol/L) | Dominant Species |
---|---|---|
0.1 | 3.8 | Cd(OH)₂(s) |
1.0 | 45.5 | [Cd(OH)₄]²⁻ |
3.0 | 116.0 | [Cd(OH)₄]²⁻ |
Data derived from NIST solubility studies . |
Reactions with Sulfides
Cadmium hydroxide reacts with hydrogen sulfide (H₂S) to form cadmium sulfide (CdS):
Cd(OH)2+H2S→CdS↓+2H2O[3]
The yellow-orange CdS precipitate is insoluble in water but dissolves in hot dilute HNO₃ or concentrated HCl .
Electrochemical Behavior
In nickel-cadmium batteries, Cd(OH)₂ participates in reversible redox reactions during discharge:
Cd+2NiO(OH)+2H2O↔Cd(OH)2+2Ni(OH)2[1][10]
This reaction underpins its use as an anode material, where Cd(OH)₂ acts as a charge-storage intermediate .
Stability and Solubility Product
The solubility product (Ksp) of Cd(OH)₂ is temperature-dependent:
Table 2: Ksp of Cd(OH)₂
Temperature (°C) | Ksp |
---|---|
25 | 7.2×10−15 |
50 | 1.1×10−13 |
Data from NIST and IUPAC studies. |
The low Ksp indicates limited dissociation in neutral water, consistent with its weak amphoteric character.
Scientific Research Applications
Cadmium hydroxide [Cd(OH)₂] has garnered attention as a promising material for supercapacitor electrodes because of its electrochemical characteristics . This has led to research and development into its potential uses in various applications, including supercapacitors, non-enzyme sensing, and more .
Scientific Applications of Cadmium Hydroxide
- Supercapacitor electrodes Cadmium hydroxide is recognized as a promising material for supercapacitor electrodes because of its unique electrochemical characteristics . Cost-effective chemical bath deposition (CBD) methods have been employed to synthesize Cd(OH)₂ nanowires on stainless-steel substrates at room temperature for use as a supercapacitor electrode . One-dimensional cadmium hydroxide nanowires have been shown to achieve a specific capacitance of 276 Fg⁻¹ at a scan rate of 5 mVs⁻¹ .
- Nanocomposites Cadmium hydroxide can be used to create nanocomposites. Mohamed Khairy et al. have documented a significant achievement in the large-scale production of CdO/Cd(OH)₂ nanocomposites, which exhibit promise for non-enzyme sensing and supercapacitor applications, demonstrating a specific capacitance of 145 F g⁻¹ at a discharge current of 2.0 A g⁻¹ .
- Precursor to Cadmium Oxide Cadmium hydroxide is a precursor to cadmium oxide . Cadmium oxide is an n-type semiconductor used in cadmium plating baths, electrodes for storage batteries, catalysts, and ceramic glazes . Cadmium oxide and cadmium telluride in the form of thin films have been used in applications such as photodiodes, phototransistors, photovoltaic solar cells, transparent electrodes, and anti-reflection coatings .
- Synthesis of Nanobelts Cadmium hydroxide can be synthesized into nanobelts using a polyol method .
Safety Considerations
Cadmium compounds are often used in coloring borosilicate glass and in lampworking . Cadmium is also produced in the manufacturing of Ni-Cd batteries . However, because of its toxicity, the use of cadmium is decreasing, and Ni-Cd batteries have been replaced with nickel-metal hydride and lithium-ion batteries .
Exposure to cadmium can result in several liver and kidney diseases . Cadmium induces various epigenetic changes in mammalian cells, both in vivo and in vitro, causing pathogenic risks and the development of various types of cancers .
Mechanism of Action
In nickel-cadmium batteries, cadmium hydroxide plays a crucial role during the discharge process. It is converted back to cadmium oxide, generating electrical energy. The reaction is as follows: [ \text{Cd(OH)}_2 + 2\text{e}^- \rightarrow \text{Cd} + 2\text{OH}^- ] This conversion is essential for the battery’s functionality and efficiency .
Comparison with Similar Compounds
Cadmium hydroxide shares structural and chemical similarities with other transition metal hydroxides, such as zinc hydroxide (Zn(OH)₂) , magnesium hydroxide (Mg(OH)₂) , and nickel hydroxide (Ni(OH)₂) . However, critical differences arise in solubility, reactivity, environmental behavior, and applications.
Solubility and Chloride Interactions
In saline environments, Cd(OH)₂ and Zn(OH)₂ exhibit distinct behaviors. Both form hydroxo-chloride complexes (e.g., CdOHCl, Zn₅(OH)₈Cl₂) in chloride-rich solutions (>0.1 mol/kg NaCl), reducing their solubility by an order of magnitude compared to pure hydroxides . However, Cd(OH)₂ is less amphoteric than Zn(OH)₂, which dissolves readily in strong bases (e.g., NaOH) to form [Zn(OH)₄]²⁻, whereas Cd(OH)₂ requires ammonia for dissolution .
Property | Cd(OH)₂ | Zn(OH)₂ | Mg(OH)₂ |
---|---|---|---|
Solubility in water | 0.13 g/100 mL | 0.42 g/100 mL | 0.0064 g/100 mL |
Amphoterism | Weakly basic | Amphoteric | Strongly basic |
Chloride interaction | Forms CdOHCl | Forms Zn₅(OH)₈Cl₂ | No stable chloride complex |
Thermal decomposition product | CdO (130–150°C) | ZnO (>125°C) | MgO (>350°C) |
Environmental Remediation
Cd(OH)₂ is less effective in soil remediation compared to Mg(OH)₂ or calcium hydroxide (Ca(OH)₂). For instance, oyster shell-derived CaCO₃ outperforms Cd(OH)₂ in immobilizing cadmium in contaminated soils due to its higher alkalinity and ion-exchange capacity . Mg(OH)₂, with its layered structure, shows superior adsorption of Cd²⁺ ions via interfacial chemical coupling, achieving >90% removal efficiency in aqueous systems .
Reactivity with CO₂ and Ligands
Binuclear Cd(II) hydroxide complexes, such as Cd₂(μ-OH)₂(bmnpa)₂₂, react with CO₂ to form carbonate bridges, a behavior analogous to zinc hydroxide complexes. However, the cadmium complex exhibits slower reaction kinetics due to its larger ionic radius and weaker Lewis acidity compared to Zn²⁺ .
Key Research Findings
- Synthesis Advancements: Hydrothermal methods yield Cd(OH)₂ nanobelts with high aspect ratios (~100 nm wide, several μm long), ideal for optoelectronic applications .
- Environmental Toxicity: Cd(OH)₂ nanoparticles show higher bioaccumulation in plants compared to Zn(OH)₂, necessitating careful disposal protocols .
- Photocatalytic Potential: Layered double hydroxides (LDHs) like MgAl-LDH outperform Cd(OH)₂ in Cr(VI) removal due to synergistic adsorption-photocatalysis .
Q & A
Q. What are the standard laboratory methods for synthesizing cadmium hydroxide, and how do reaction conditions influence purity?
Cadmium hydroxide (Cd(OH)₂) is typically synthesized via precipitation reactions. A common method involves adding sodium hydroxide (NaOH) to cadmium nitrate or cadmium chloride solutions under controlled pH (8–10) and temperature (25–100°C) . For example:
- Nitrate route : Cd(NO₃)₂ + 2NaOH → Cd(OH)₂↓ + 2NaNO₃.
- Chloride route : CdCl₂ + 2NaOH → Cd(OH)₂↓ + 2NaCl.
Key factors affecting purity :
- Temperature : Higher temperatures (>80°C) reduce residual sodium ions but may induce partial decomposition to CdO .
- Stoichiometric control : Excess NaOH can lead to soluble hydroxocadmium complexes (e.g., [Cd(OH)₃]⁻), requiring precise titration .
- Washing protocols : Repeated centrifugation with deionized water removes ionic impurities, confirmed via conductivity measurements (<10 µS/cm) .
Q. How can researchers characterize the structural and electrochemical properties of cadmium hydroxide?
Core techniques :
- XRD : Confirms crystallinity and phase purity. Cd(OH)₂ exhibits a hexagonal structure (P3₁m space group) with a basal spacing of ~4.7 Å .
- FT-IR : Peaks at 3640 cm⁻¹ (O-H stretching) and 520 cm⁻¹ (Cd-O vibrations) validate hydroxide formation .
- Cyclic Voltammetry (CV) : In 0.1 M NaOH, Cd(OH)₂ shows redox peaks at -0.9 V (reduction to Cd) and -0.7 V (oxidation to CdO), with sweep rates (0.01–20 V/s) influencing peak separation .
- TGA : Decomposition occurs in two stages: dehydration (130–200°C) and conversion to CdO (>300°C) .
Advanced methods :
- Rotating Disk Electrode (RDE) : Quantifies diffusion-controlled processes; e.g., Cd²⁺ ion transport in alkaline media under varying rotation speeds (0–2600 rpm) .
- In-situ Raman Spectroscopy : Monitors phase transitions during electrochemical cycling, critical for battery applications .
Q. What experimental designs resolve contradictions in cadmium hydroxide’s electrochemical data under non-stationary conditions?
Contradictions often arise from incomplete reduction/oxidation cycles or solution-phase intermediates. Methodological solutions :
- Potential Step Chronoamperometry : Isolates contributions from surface-bound vs. solution-phase species by applying fixed potentials (-1.76 to -0.76 V vs. SCE) and analyzing current decay kinetics .
- Modulated Voltammetry : Triangularly modulated sweeps (TMTPS) with guide ramps (0.5 V/s) differentiate capacitive currents from faradaic processes, reducing artifacts .
- Controlled Atmosphere : Purge solutions with nitrogen to eliminate oxygen interference, which can catalyze parasitic reactions (e.g., Cd(OH)₂ → CdOOH) .
Q. How can cadmium hydroxide’s adsorption capacity for heavy metals be optimized, and what models describe its kinetics?
Optimization strategies :
- Composite Design : Intercalate Cd(OH)₂ into layered double hydroxides (LDH) pillared with polyoxometalates (POMs). For example, Ca-Al/POM composites achieve 98% Cd²⁺ removal at pH 6–8 due to enhanced anion exchange capacity .
- Surface Functionalization : Graft thiol (-SH) or amine (-NH₂) groups to increase binding sites for Cd²⁺ .
Adsorption models :
- Isotherms : Freundlich model (R² > 0.95) indicates multilayer adsorption on heterogeneous surfaces, with Kf = 12.3 mg/g .
- Kinetics : Pseudo-second-order model (k₂ = 0.045 g/mg·min) suggests chemisorption dominates .
Q. What challenges arise in reproducing cadmium hydroxide synthesis from literature, and how are they addressed?
Common issues :
- Impurity carryover : Residual Na⁺/Cl⁻ from incomplete washing alters electrochemical behavior. Solution : Use dialysis membranes for extended purification .
- Phase instability : Cd(OH)₂ → CdO conversion during drying. Mitigation : Lyophilize samples at -50°C to retain hydroxyl groups .
- Inconsistent characterization : Varied XRD scan rates (e.g., 2°/min vs. 5°/min) affect peak resolution. Standardization : Adhere to ISO 20203:2018 for diffraction protocols .
Q. How do researchers validate cadmium hydroxide’s role in battery electrodes, and what metrics assess performance?
Validation protocols :
- Galvanostatic Cycling : Test Cd(OH)₂ anodes in Ni-Cd cells at C/5 rates (0.2 mA/cm²). Capacity retention >80% after 500 cycles indicates stability .
- Impedance Spectroscopy : Nyquist plots reveal charge-transfer resistance (Rct < 50 Ω·cm²) and Warburg diffusion coefficients .
- Post-mortem Analysis : SEM/EDS identifies dendrite formation or electrode cracking, common failure modes in alkaline electrolytes .
Key metrics :
- Specific Capacity : Theoretical 375 mAh/g for Cd(OH)₂ → Cd redox .
- Coulombic Efficiency : >99% indicates minimal side reactions (e.g., hydrogen evolution) .
Q. What advanced computational methods predict cadmium hydroxide’s reactivity in novel applications?
- DFT Calculations : Simulate adsorption energies (E_ads) of Cd²⁺ on hydroxide surfaces. For example, E_ads = -2.3 eV on oxygen-terminated sites suggests strong chemisorption .
- Molecular Dynamics (MD) : Model ion diffusion pathways in Cd(OH)₂ lattices, revealing activation barriers (~0.7 eV) for proton hopping .
- COMSOL Multiphysics : Predict current distribution in porous Cd(OH)₂ electrodes, optimizing thickness (100–200 µm) for minimal polarization .
Properties
Molecular Formula |
CdHO- |
---|---|
Molecular Weight |
129.42 g/mol |
IUPAC Name |
cadmium;hydroxide |
InChI |
InChI=1S/Cd.H2O/h;1H2/p-1 |
InChI Key |
HUKFCVYEXPZJJZ-UHFFFAOYSA-M |
Canonical SMILES |
[OH-].[Cd] |
Origin of Product |
United States |
Disclaimer and Information on In-Vitro Research Products
Please be aware that all articles and product information presented on BenchChem are intended solely for informational purposes. The products available for purchase on BenchChem are specifically designed for in-vitro studies, which are conducted outside of living organisms. In-vitro studies, derived from the Latin term "in glass," involve experiments performed in controlled laboratory settings using cells or tissues. It is important to note that these products are not categorized as medicines or drugs, and they have not received approval from the FDA for the prevention, treatment, or cure of any medical condition, ailment, or disease. We must emphasize that any form of bodily introduction of these products into humans or animals is strictly prohibited by law. It is essential to adhere to these guidelines to ensure compliance with legal and ethical standards in research and experimentation.