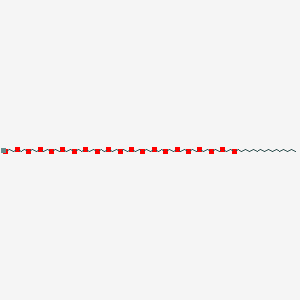
Cetomacrogol 1000
Overview
Description
Cetomacrogol 1000, also known as polyethylene glycol hexadecyl ether, is a non-ionic surfactant widely used in pharmaceutical and cosmetic formulations. It is produced by the condensation of cetyl alcohol with ethylene oxide under controlled conditions to achieve the desired molecular structure. This compound is known for its excellent emulsifying and solubilizing properties, making it a valuable ingredient in creams, lotions, and other topical applications .
Mechanism of Action
Target of Action
Cetomacrogol 1000, also known as polyethylene glycol hexadecyl ether, is a nonionic surfactant . It is primarily used as an emulsifier and solubilizer in various industries, including food, cosmetics, and pharmaceuticals . Its primary targets are essential oils, vitamin oils, and drugs with low water solubility .
Mode of Action
This compound acts as an oil-in-water (O/W) emulsifier . It works by reducing the surface tension between two immiscible (unmixable) substances, such as oil and water, allowing them to form a stable, homogenous mixture . This is particularly useful in the formulation of creams and lotions, where it helps to evenly distribute the active ingredients .
Biochemical Pathways
For instance, by solubilizing essential oils or drugs, it can enhance their dispersion in aqueous solutions, potentially improving their absorption and distribution in the body .
Pharmacokinetics
As a topical agent, it is generally considered to have minimal systemic absorption . Its primary function is to improve the delivery and absorption of other substances at the site of application .
Result of Action
The main result of this compound’s action is the formation of stable emulsions, which can enhance the delivery and efficacy of other substances . By solubilizing oils and drugs, it can improve their dispersion in aqueous solutions, potentially enhancing their bioavailability and therapeutic effects .
Action Environment
This compound is stable under moderately strong acid and alkaline conditions . This stability allows it to maintain its emulsifying and solubilizing properties in various environments, making it a versatile ingredient in many formulations . The exact influence of environmental factors on its action, efficacy, and stability may depend on the specific formulation and conditions of use .
Biochemical Analysis
Biochemical Properties
Cetomacrogol 1000 acts as an oil-in-water (O/W) emulsifier and solubilizer for essential oils, vitamin oils, and drugs of low water solubility . It is stable under moderately strong acid and alkaline conditions
Cellular Effects
It has been suggested that it may cause hemolysis, a process where red blood cells rupture and release their contents into surrounding fluid . This process is believed to involve the formation of peroxides and the hydroxyl radical .
Molecular Mechanism
This compound is produced by the ethoxylation of cetyl alcohol, resulting in a compound with the general formula HO (C 2 H 4 O) n C 16 H 33 .
Temporal Effects in Laboratory Settings
This compound is known to be stable under moderately strong acid and alkaline conditions
Preparation Methods
Synthetic Routes and Reaction Conditions
The synthesis of Cetomacrogol 1000 involves the reaction of cetyl alcohol with ethylene oxide. The process is typically carried out under controlled conditions to ensure the desired degree of ethoxylation. The reaction can be represented as follows:
C16H33OH+n(CH2CH2O)→C16H33(OCH2CH2)nOH
where ( n ) represents the number of ethylene oxide units added to the cetyl alcohol molecule .
Industrial Production Methods
In industrial settings, the production of this compound involves the use of large-scale reactors where cetyl alcohol and ethylene oxide are combined under controlled temperature and pressure conditions. The reaction is typically catalyzed by an alkaline catalyst, such as sodium hydroxide or potassium hydroxide. The resulting product is then purified and processed into a waxy solid form, which can be used in various formulations .
Chemical Reactions Analysis
Types of Reactions
Cetomacrogol 1000 primarily undergoes reactions typical of non-ionic surfactants. These include:
Oxidation: this compound can be oxidized under specific conditions, leading to the formation of various oxidation products.
Reduction: Reduction reactions are less common but can occur under certain conditions.
Substitution: This compound can participate in substitution reactions, particularly in the presence of reactive agents.
Common Reagents and Conditions
Oxidation: Common oxidizing agents include hydrogen peroxide and potassium permanganate.
Reduction: Reducing agents such as sodium borohydride can be used.
Substitution: Various halogenating agents and other reactive chemicals can facilitate substitution reactions.
Major Products Formed
The major products formed from these reactions depend on the specific conditions and reagents used. For example, oxidation can lead to the formation of aldehydes, ketones, and carboxylic acids .
Scientific Research Applications
Cetomacrogol 1000 has a wide range of applications in scientific research, including:
Chemistry: Used as an emulsifier and solubilizer in various chemical formulations.
Biology: Employed in the preparation of biological samples and as a stabilizing agent in biochemical assays.
Medicine: Utilized in pharmaceutical formulations for topical applications, such as creams and lotions.
Industry: Applied in the production of cosmetics, personal care products, and other industrial formulations
Comparison with Similar Compounds
Similar Compounds
Polyethylene glycol stearate: Another non-ionic surfactant with similar emulsifying properties.
Polyethylene glycol laurate: Known for its solubilizing capabilities.
Polyethylene glycol oleate: Used in various cosmetic and pharmaceutical formulations.
Uniqueness of Cetomacrogol 1000
This compound is unique due to its specific balance of hydrophilic and hydrophobic properties, which makes it particularly effective in stabilizing oil-in-water emulsions. Its ability to form stable emulsions under a wide range of pH conditions and its compatibility with various active ingredients make it a versatile and valuable ingredient in many formulations .
Properties
IUPAC Name |
2-[2-[2-[2-[2-[2-[2-[2-[2-[2-[2-[2-[2-[2-[2-[2-[2-[2-[2-(2-hexadecoxyethoxy)ethoxy]ethoxy]ethoxy]ethoxy]ethoxy]ethoxy]ethoxy]ethoxy]ethoxy]ethoxy]ethoxy]ethoxy]ethoxy]ethoxy]ethoxy]ethoxy]ethoxy]ethoxy]ethanol | |
---|---|---|
Source | PubChem | |
URL | https://pubchem.ncbi.nlm.nih.gov | |
Description | Data deposited in or computed by PubChem | |
InChI |
InChI=1S/C56H114O21/c1-2-3-4-5-6-7-8-9-10-11-12-13-14-15-17-58-19-21-60-23-25-62-27-29-64-31-33-66-35-37-68-39-41-70-43-45-72-47-49-74-51-53-76-55-56-77-54-52-75-50-48-73-46-44-71-42-40-69-38-36-67-34-32-65-30-28-63-26-24-61-22-20-59-18-16-57/h57H,2-56H2,1H3 | |
Source | PubChem | |
URL | https://pubchem.ncbi.nlm.nih.gov | |
Description | Data deposited in or computed by PubChem | |
InChI Key |
NLMKTBGFQGKQEV-UHFFFAOYSA-N | |
Source | PubChem | |
URL | https://pubchem.ncbi.nlm.nih.gov | |
Description | Data deposited in or computed by PubChem | |
Canonical SMILES |
CCCCCCCCCCCCCCCCOCCOCCOCCOCCOCCOCCOCCOCCOCCOCCOCCOCCOCCOCCOCCOCCOCCOCCOCCOCCO | |
Source | PubChem | |
URL | https://pubchem.ncbi.nlm.nih.gov | |
Description | Data deposited in or computed by PubChem | |
Molecular Formula |
C56H114O21 | |
Source | PubChem | |
URL | https://pubchem.ncbi.nlm.nih.gov | |
Description | Data deposited in or computed by PubChem | |
DSSTOX Substance ID |
DTXSID7046708 | |
Record name | Polyethylene glycol (20) hexadecyl ether | |
Source | EPA DSSTox | |
URL | https://comptox.epa.gov/dashboard/DTXSID7046708 | |
Description | DSSTox provides a high quality public chemistry resource for supporting improved predictive toxicology. | |
Molecular Weight |
1123.5 g/mol | |
Source | PubChem | |
URL | https://pubchem.ncbi.nlm.nih.gov | |
Description | Data deposited in or computed by PubChem | |
Physical Description |
White solid; mp = 32-34 deg C; [Sigma-Aldrich MSDS] | |
Record name | Cetomacrogol | |
Source | Haz-Map, Information on Hazardous Chemicals and Occupational Diseases | |
URL | https://haz-map.com/Agents/12872 | |
Description | Haz-Map® is an occupational health database designed for health and safety professionals and for consumers seeking information about the adverse effects of workplace exposures to chemical and biological agents. | |
Explanation | Copyright (c) 2022 Haz-Map(R). All rights reserved. Unless otherwise indicated, all materials from Haz-Map are copyrighted by Haz-Map(R). No part of these materials, either text or image may be used for any purpose other than for personal use. Therefore, reproduction, modification, storage in a retrieval system or retransmission, in any form or by any means, electronic, mechanical or otherwise, for reasons other than personal use, is strictly prohibited without prior written permission. | |
CAS No. |
9004-95-9 | |
Record name | Cetomacrogol | |
Source | ChemIDplus | |
URL | https://pubchem.ncbi.nlm.nih.gov/substance/?source=chemidplus&sourceid=0009004959 | |
Description | ChemIDplus is a free, web search system that provides access to the structure and nomenclature authority files used for the identification of chemical substances cited in National Library of Medicine (NLM) databases, including the TOXNET system. | |
Record name | Poly(oxy-1,2-ethanediyl), .alpha.-hexadecyl-.omega.-hydroxy- | |
Source | EPA Chemicals under the TSCA | |
URL | https://www.epa.gov/chemicals-under-tsca | |
Description | EPA Chemicals under the Toxic Substances Control Act (TSCA) collection contains information on chemicals and their regulations under TSCA, including non-confidential content from the TSCA Chemical Substance Inventory and Chemical Data Reporting. | |
Record name | Hexadecan- l-ol, ethoxylated | |
Source | European Chemicals Agency (ECHA) | |
URL | https://echa.europa.eu/substance-information/-/substanceinfo/100.105.524 | |
Description | The European Chemicals Agency (ECHA) is an agency of the European Union which is the driving force among regulatory authorities in implementing the EU's groundbreaking chemicals legislation for the benefit of human health and the environment as well as for innovation and competitiveness. | |
Explanation | Use of the information, documents and data from the ECHA website is subject to the terms and conditions of this Legal Notice, and subject to other binding limitations provided for under applicable law, the information, documents and data made available on the ECHA website may be reproduced, distributed and/or used, totally or in part, for non-commercial purposes provided that ECHA is acknowledged as the source: "Source: European Chemicals Agency, http://echa.europa.eu/". Such acknowledgement must be included in each copy of the material. ECHA permits and encourages organisations and individuals to create links to the ECHA website under the following cumulative conditions: Links can only be made to webpages that provide a link to the Legal Notice page. | |
Disclaimer and Information on In-Vitro Research Products
Please be aware that all articles and product information presented on BenchChem are intended solely for informational purposes. The products available for purchase on BenchChem are specifically designed for in-vitro studies, which are conducted outside of living organisms. In-vitro studies, derived from the Latin term "in glass," involve experiments performed in controlled laboratory settings using cells or tissues. It is important to note that these products are not categorized as medicines or drugs, and they have not received approval from the FDA for the prevention, treatment, or cure of any medical condition, ailment, or disease. We must emphasize that any form of bodily introduction of these products into humans or animals is strictly prohibited by law. It is essential to adhere to these guidelines to ensure compliance with legal and ethical standards in research and experimentation.