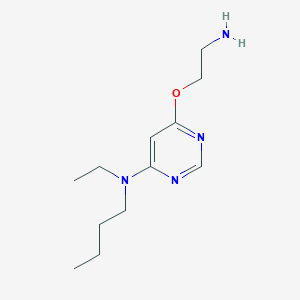
6-(2-aminoethoxy)-N-butyl-N-ethylpyrimidin-4-amine
Overview
Description
The compound “6-(2-aminoethoxy)-N-butyl-N-ethylpyrimidin-4-amine” is a pyrimidine derivative. Pyrimidines are aromatic heterocyclic organic compounds similar to benzene and pyridine, containing two nitrogen atoms at positions 1 and 3 of the six-member ring .
Molecular Structure Analysis
The molecular structure of this compound, based on its name, would contain a pyrimidine ring with an aminoethoxy group at the 6-position and butyl and ethyl groups attached to a nitrogen atom. The exact structure and its properties would depend on the specific locations of these substituents .Chemical Reactions Analysis
As a pyrimidine derivative, this compound might undergo reactions similar to other pyrimidines, which can include electrophilic and nucleophilic substitutions, and reactions at the nitrogen atoms .Scientific Research Applications
Amination of Pyrimidines
Research on the amination of pyrimidine derivatives, such as the study by Rasmussen and Plas, explores the reactions of halogenated pyrimidines with potassium amide in liquid ammonia. This process is fundamental in the synthesis of amino-substituted pyrimidines, which are crucial intermediates in pharmaceuticals and agrochemicals (Rasmussen & Plas, 2010).
Synthesis of Aminopyrimidines
The synthesis of 4-aryloxy-6-methylpyrimidin-2-amines by Erkin et al. involves the reaction of 2-amino-6-methylpyrimidin-4-yl 4-methylbenzenesulfonate with phenols. This method contributes to the development of compounds with potential biological activities (Erkin et al., 2015).
Ligands for Histamine H4 Receptors
Altenbach et al. conducted structure-activity studies on a series of 2-aminopyrimidine-containing ligands for the histamine H4 receptor, which is implicated in inflammatory and immune responses. This research aids in understanding the role of pyrimidine derivatives in modulating histamine-mediated pathways (Altenbach et al., 2008).
Analgesic and Anti-inflammatory Agents
Chhabria et al. synthesized and evaluated 5-ethoxycarbonyl-6-isopropylamino-4-(substitutedphenyl)aminopyrimidines for their analgesic and anti-inflammatory properties. Such studies highlight the therapeutic potential of pyrimidine derivatives (Chhabria et al., 2007).
Photoredox Catalysis
Ociepa et al. presented a metal-free photoredox strategy using redox-activated primary amine derivatives for forming C(sp3)–C(sp) and C(sp3)–C(sp2) bonds. This research underscores the utility of pyrimidine-based compounds in catalysis and organic synthesis (Ociepa et al., 2018).
Adsorption Studies
Garcia-Martin et al. explored the use of a pyrimidine-polyamine conjugate as an ion receptor for Zn2+ and Cd2+ ions in aqueous solutions. This research provides insights into the environmental applications of pyrimidine derivatives for heavy metal removal (Garcia-Martin et al., 2005).
EGFR Tyrosine Kinase Inhibition
Kaspersen et al. synthesized 4-N-substituted 6-aryl-7H-pyrrolo[2,3-d]pyrimidine-4-amines and tested them for their in vitro EGFR (ErbB1) tyrosine kinase inhibitory activity, which is relevant in cancer therapy (Kaspersen et al., 2011).
Mechanism of Action
Target of Action
The primary target of 6-(2-aminoethoxy)-N-butyl-N-ethylpyrimidin-4-amine, also known as Istaroxime, is the sodium/potassium adenosine triphosphatase (Na+/K+ ATPase) . This enzyme plays a crucial role in maintaining the electrochemical gradient across the cell membrane, which is essential for numerous physiological processes.
Mode of Action
Istaroxime inhibits the activity of Na+/K+ ATPase . This inhibition increases intracellular sodium levels, which reverses the driving force of the sodium/calcium exchanger, inhibiting calcium extrusion and possibly facilitating calcium entry .
Biochemical Pathways
The inhibition of Na+/K+ ATPase and the subsequent increase in intracellular sodium levels affect the sodium/calcium exchanger, leading to changes in intracellular calcium concentrations . This can enhance the efficacy by which intracellular calcium triggers sarcoplasmic reticulum calcium release . It also accelerates the inactivation state of L-type calcium channels, which allow for calcium influx . These changes in calcium handling increase cell contraction .
Result of Action
The result of the action of 6-(2-aminoethoxy)-N-butyl-N-ethylpyrimidin-4-amine is an increase in cell contraction due to changes in calcium handling . It also enhances the heart’s relaxation phase by increasing the rate of intracellular calcium sequestration by Sarco/endoplasmic Reticulum Calcium ATPase, isotype 2a (SERCA2a) .
properties
IUPAC Name |
6-(2-aminoethoxy)-N-butyl-N-ethylpyrimidin-4-amine | |
---|---|---|
Source | PubChem | |
URL | https://pubchem.ncbi.nlm.nih.gov | |
Description | Data deposited in or computed by PubChem | |
InChI |
InChI=1S/C12H22N4O/c1-3-5-7-16(4-2)11-9-12(15-10-14-11)17-8-6-13/h9-10H,3-8,13H2,1-2H3 | |
Source | PubChem | |
URL | https://pubchem.ncbi.nlm.nih.gov | |
Description | Data deposited in or computed by PubChem | |
InChI Key |
CAINBCNMMLKJDV-UHFFFAOYSA-N | |
Source | PubChem | |
URL | https://pubchem.ncbi.nlm.nih.gov | |
Description | Data deposited in or computed by PubChem | |
Canonical SMILES |
CCCCN(CC)C1=CC(=NC=N1)OCCN | |
Source | PubChem | |
URL | https://pubchem.ncbi.nlm.nih.gov | |
Description | Data deposited in or computed by PubChem | |
Molecular Formula |
C12H22N4O | |
Source | PubChem | |
URL | https://pubchem.ncbi.nlm.nih.gov | |
Description | Data deposited in or computed by PubChem | |
Molecular Weight |
238.33 g/mol | |
Source | PubChem | |
URL | https://pubchem.ncbi.nlm.nih.gov | |
Description | Data deposited in or computed by PubChem | |
Product Name |
6-(2-aminoethoxy)-N-butyl-N-ethylpyrimidin-4-amine |
Retrosynthesis Analysis
AI-Powered Synthesis Planning: Our tool employs the Template_relevance Pistachio, Template_relevance Bkms_metabolic, Template_relevance Pistachio_ringbreaker, Template_relevance Reaxys, Template_relevance Reaxys_biocatalysis model, leveraging a vast database of chemical reactions to predict feasible synthetic routes.
One-Step Synthesis Focus: Specifically designed for one-step synthesis, it provides concise and direct routes for your target compounds, streamlining the synthesis process.
Accurate Predictions: Utilizing the extensive PISTACHIO, BKMS_METABOLIC, PISTACHIO_RINGBREAKER, REAXYS, REAXYS_BIOCATALYSIS database, our tool offers high-accuracy predictions, reflecting the latest in chemical research and data.
Strategy Settings
Precursor scoring | Relevance Heuristic |
---|---|
Min. plausibility | 0.01 |
Model | Template_relevance |
Template Set | Pistachio/Bkms_metabolic/Pistachio_ringbreaker/Reaxys/Reaxys_biocatalysis |
Top-N result to add to graph | 6 |
Feasible Synthetic Routes
Disclaimer and Information on In-Vitro Research Products
Please be aware that all articles and product information presented on BenchChem are intended solely for informational purposes. The products available for purchase on BenchChem are specifically designed for in-vitro studies, which are conducted outside of living organisms. In-vitro studies, derived from the Latin term "in glass," involve experiments performed in controlled laboratory settings using cells or tissues. It is important to note that these products are not categorized as medicines or drugs, and they have not received approval from the FDA for the prevention, treatment, or cure of any medical condition, ailment, or disease. We must emphasize that any form of bodily introduction of these products into humans or animals is strictly prohibited by law. It is essential to adhere to these guidelines to ensure compliance with legal and ethical standards in research and experimentation.