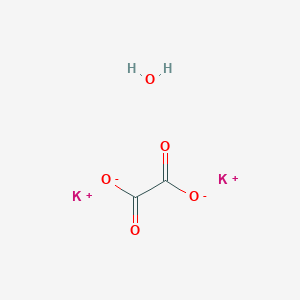
Potassium oxalate monohydrate
Overview
Description
Potassium oxalate monohydrate is a chemical compound with the formula (K_2C_2O_4 \cdot H_2O). It is the monohydrate form of potassium oxalate, a salt of oxalic acid. This compound appears as white, odorless crystals that are highly soluble in water. This compound is commonly used in various industrial and scientific applications due to its chelating properties and its ability to form complexes with metal ions .
Mechanism of Action
Target of Action
Potassium oxalate monohydrate (POM) primarily targets calcium ions in the body . It forms insoluble calcium oxalate crystals, which are the main constituents of kidney stones . POM also interacts with hydrogen ions in aqueous solutions, acting as a base to neutralize acids .
Mode of Action
POM interacts with its targets through chelation and precipitation . It binds with calcium ions to form insoluble calcium oxalate . This interaction is crucial in the formation of kidney stones . In aqueous solutions, POM can react as a base to neutralize acids, generating heat and carbon dioxide .
Biochemical Pathways
POM affects several biochemical pathways. It plays a role in the biogeochemical cycles of certain nutrients and influences their bioavailability . POM is also involved in the oxalate-carbonate pathway , which is an effective way for the transfer of atmospheric carbon dioxide into calcium carbonate as a carbon reservoir .
Pharmacokinetics
It is known that pom is soluble in water , which could influence its bioavailability and distribution in the body.
Result of Action
The primary result of POM’s action is the formation of calcium oxalate crystals . These crystals can cause injury to renal epithelial cells, leading to conditions like nephrolithiasis (kidney stones) . The cytotoxicity of these crystals is closely related to their morphology and surface structure .
Action Environment
The action of POM is influenced by various environmental factors. For instance, the availability of carbon and nitrogen sources, presence of metal oxalates, and pH can affect the production of oxalic acid by fungi . Additionally, the concentration of calcium ions in the body can impact the formation of calcium oxalate crystals .
Biochemical Analysis
Biochemical Properties
Potassium oxalate monohydrate plays a significant role in biochemical reactions, particularly in the context of calcium oxalate formation. It interacts with calcium ions to form calcium oxalate, a process that is crucial in the study of kidney stones. The compound also interacts with enzymes such as oxalate oxidase, which catalyzes the oxidation of oxalate to carbon dioxide and hydrogen peroxide. This interaction is essential in understanding the metabolic pathways involving oxalate and its role in various physiological processes .
Cellular Effects
This compound affects various types of cells and cellular processes. In renal epithelial cells, it can induce oxidative stress and inflammation, leading to cell damage and apoptosis. This compound influences cell signaling pathways, including the activation of nuclear factor kappa-light-chain-enhancer of activated B cells (NF-κB) and mitogen-activated protein kinases (MAPKs). These pathways are involved in the regulation of gene expression and cellular metabolism, highlighting the impact of this compound on cell function .
Molecular Mechanism
The molecular mechanism of this compound involves its interaction with biomolecules such as proteins and enzymes. It binds to calcium ions, forming insoluble calcium oxalate crystals, which can lead to cellular damage and inflammation. Additionally, this compound can inhibit the activity of certain enzymes, such as oxalate decarboxylase, which is involved in the degradation of oxalate. This inhibition can result in the accumulation of oxalate and subsequent cellular effects .
Temporal Effects in Laboratory Settings
In laboratory settings, the effects of this compound can change over time. The compound is relatively stable under standard conditions, but it can degrade in the presence of strong acids or bases. Long-term exposure to this compound in in vitro studies has shown that it can lead to chronic oxidative stress and inflammation in cells, affecting cellular function and viability .
Dosage Effects in Animal Models
The effects of this compound vary with different dosages in animal models. At low doses, it may not cause significant adverse effects, but at higher doses, it can induce toxicity, leading to renal damage and the formation of kidney stones. Threshold effects have been observed, where a certain dosage level results in a marked increase in adverse outcomes. High doses of this compound can also lead to systemic toxicity, affecting multiple organs .
Metabolic Pathways
This compound is involved in metabolic pathways related to oxalate metabolism. It interacts with enzymes such as oxalate oxidase and oxalate decarboxylase, which are responsible for the degradation of oxalate. These interactions can affect metabolic flux and the levels of metabolites such as carbon dioxide and formate. The compound’s role in these pathways is crucial for understanding its impact on metabolic processes and its potential therapeutic applications .
Transport and Distribution
Within cells and tissues, this compound is transported and distributed through various mechanisms. It can be taken up by cells via specific transporters or through passive diffusion. Once inside the cell, it may interact with binding proteins that influence its localization and accumulation. The distribution of this compound within tissues can affect its biological activity and potential toxicity .
Subcellular Localization
This compound’s subcellular localization is influenced by targeting signals and post-translational modifications. It can be directed to specific compartments or organelles, such as the mitochondria or lysosomes, where it exerts its effects. The localization of this compound within these subcellular structures can impact its activity and function, contributing to its overall biochemical properties .
Preparation Methods
Synthetic Routes and Reaction Conditions: Potassium oxalate monohydrate can be synthesized by reacting oxalic acid with potassium hydroxide. The reaction typically occurs in an aqueous solution, and the product is then crystallized from the solution. The reaction can be represented as follows: [ \text{H}_2\text{C}_2\text{O}_4 + 2\text{KOH} \rightarrow \text{K}_2\text{C}_2\text{O}_4 + 2\text{H}_2\text{O} ]
Industrial Production Methods: In industrial settings, this compound is produced by neutralizing oxalic acid with potassium carbonate or potassium hydroxide. The resulting solution is then evaporated to obtain the crystalline product. The process involves careful control of temperature and pH to ensure high purity and yield .
Types of Reactions:
Oxidation: this compound can undergo oxidation reactions, where it is converted to potassium carbonate and carbon dioxide.
Reduction: It can act as a reducing agent in certain chemical reactions, such as the reduction of metal ions.
Complexation: this compound forms complexes with various metal ions, which is useful in analytical chemistry.
Common Reagents and Conditions:
Oxidizing Agents: Potassium permanganate or hydrogen peroxide can be used as oxidizing agents.
Reducing Agents: It can reduce metal ions in the presence of acids.
Complexation Conditions: Typically involves aqueous solutions and controlled pH levels.
Major Products Formed:
Oxidation: Potassium carbonate and carbon dioxide.
Reduction: Reduced metal ions and oxalic acid.
Complexation: Metal-oxalate complexes
Scientific Research Applications
Potassium oxalate monohydrate has a wide range of applications in scientific research:
Chemistry: Used as a reagent in analytical chemistry for the determination of calcium and other heavy metals. It is also used in the preparation of catalysts and in various synthetic reactions.
Biology: Employed in studies involving metal ion chelation and its effects on biological systems.
Medicine: Investigated for its potential use in medical treatments involving metal ion chelation.
Industry: Utilized as a bleaching agent in the textile and wood industries, a rust remover in metal treatment, and a scale remover in automobile radiators. .
Comparison with Similar Compounds
Ammonium oxalate monohydrate: Similar in structure and properties, but uses ammonium ions instead of potassium ions.
Sodium oxalate: Another oxalate salt, but with sodium ions.
Calcium oxalate: Commonly found in kidney stones, less soluble in water compared to potassium oxalate.
Uniqueness of Potassium Oxalate Monohydrate: this compound is unique due to its high solubility in water and its ability to form stable complexes with a wide range of metal ions. This makes it particularly useful in applications requiring precise control of metal ion concentrations .
Properties
CAS No. |
6487-48-5 |
---|---|
Molecular Formula |
C2H4KO5 |
Molecular Weight |
147.15 g/mol |
IUPAC Name |
dipotassium;oxalate;hydrate |
InChI |
InChI=1S/C2H2O4.K.H2O/c3-1(4)2(5)6;;/h(H,3,4)(H,5,6);;1H2 |
InChI Key |
RKKPSAKHSRTUBZ-UHFFFAOYSA-N |
SMILES |
C(=O)(C(=O)[O-])[O-].O.[K+].[K+] |
Canonical SMILES |
C(=O)(C(=O)O)O.O.[K] |
Pictograms |
Irritant |
Related CAS |
6100-20-5 |
Synonyms |
K2C2O4.H2O, Ethanedioic acid dipotassium salt monohydrate |
Origin of Product |
United States |
Retrosynthesis Analysis
AI-Powered Synthesis Planning: Our tool employs the Template_relevance Pistachio, Template_relevance Bkms_metabolic, Template_relevance Pistachio_ringbreaker, Template_relevance Reaxys, Template_relevance Reaxys_biocatalysis model, leveraging a vast database of chemical reactions to predict feasible synthetic routes.
One-Step Synthesis Focus: Specifically designed for one-step synthesis, it provides concise and direct routes for your target compounds, streamlining the synthesis process.
Accurate Predictions: Utilizing the extensive PISTACHIO, BKMS_METABOLIC, PISTACHIO_RINGBREAKER, REAXYS, REAXYS_BIOCATALYSIS database, our tool offers high-accuracy predictions, reflecting the latest in chemical research and data.
Strategy Settings
Precursor scoring | Relevance Heuristic |
---|---|
Min. plausibility | 0.01 |
Model | Template_relevance |
Template Set | Pistachio/Bkms_metabolic/Pistachio_ringbreaker/Reaxys/Reaxys_biocatalysis |
Top-N result to add to graph | 6 |
Feasible Synthetic Routes
Q1: What is the molecular formula and weight of potassium oxalate monohydrate?
A1: this compound has the molecular formula K2C2O4·H2O and a molecular weight of 184.22 g/mol.
Q2: What spectroscopic techniques are useful for characterizing this compound?
A2: Several spectroscopic techniques have been employed to study this compound, including:
- Infrared (IR) spectroscopy: Useful for identifying functional groups and studying hydrogen bonding. [, ]
- Raman spectroscopy: Complementary to IR, providing information about molecular vibrations. [, ]
- Nuclear Magnetic Resonance (NMR) spectroscopy: Proton and deuterium NMR have been used to study the motion and environment of water molecules within the crystal structure. [, , , , ]
- Electron Paramagnetic Resonance (EPR) spectroscopy: Used to investigate the electronic structure and local environment of paramagnetic impurities like Cu2+ and VO2+ doped into this compound crystals. [, , , ]
Q3: Is this compound thermally stable?
A3: this compound undergoes dehydration upon heating. Differential thermal analysis and thermogravimetric analysis have revealed the existence of three anhydrous polymorphs of potassium oxalate, with phase transitions occurring at specific temperatures. []
Q4: What is known about the motion of water molecules within the crystal structure of this compound?
A4: Research using deuterium NMR and inelastic neutron scattering has shown that the water molecules in this compound undergo a 180° flipping motion. The energy barrier for this motion has been determined, and the angular amplitudes of torsional and rocking motions have been estimated. [, , ]
Q5: What are some applications of this compound?
A5: this compound has found use in various applications:
- Fire suppression: It exhibits good fire extinguishing efficiency and reignition resistance, particularly when combined with sodium bicarbonate. []
- Preparation of oxaliplatin: It serves as a reagent in the synthesis of the anti-tumor drug oxaliplatin. [, ]
- Production of porous carbons: It can be used as an activation agent for preparing porous carbon materials with high surface areas, desirable for applications like electric double-layer capacitors. [, ]
Q6: How does this compound contribute to the synthesis of oxaliplatin?
A6: In the synthesis of oxaliplatin, this compound reacts with a platinum(II) precursor to form a key intermediate, bis(oxalato)platinum(II). This intermediate then reacts with (1R,2R)-(-)-1,2-cyclohexanediamine to yield oxaliplatin. [, ]
Q7: What are the advantages of using this compound in the synthesis of porous carbons?
A7: this compound, when used as an activation agent, contributes to the formation of nitrogen- and oxygen-doped porous carbons with high surface areas. These heteroatom-doped carbons exhibit enhanced performance in applications like electric double-layer capacitors due to their improved electrical conductivity and wettability. []
Q8: Have there been any computational studies on this compound?
A8: Yes, density functional theory (DFT) calculations have been employed to study the electronic structure and properties of this compound. These calculations have been used to investigate the 17O quadrupolar coupling constants and chemical shift tensors of water molecules within the crystal structure. []
Disclaimer and Information on In-Vitro Research Products
Please be aware that all articles and product information presented on BenchChem are intended solely for informational purposes. The products available for purchase on BenchChem are specifically designed for in-vitro studies, which are conducted outside of living organisms. In-vitro studies, derived from the Latin term "in glass," involve experiments performed in controlled laboratory settings using cells or tissues. It is important to note that these products are not categorized as medicines or drugs, and they have not received approval from the FDA for the prevention, treatment, or cure of any medical condition, ailment, or disease. We must emphasize that any form of bodily introduction of these products into humans or animals is strictly prohibited by law. It is essential to adhere to these guidelines to ensure compliance with legal and ethical standards in research and experimentation.