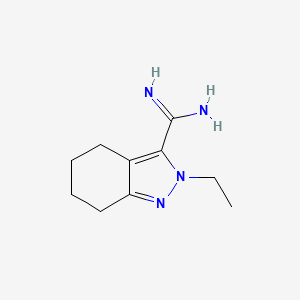
2-ethyl-4,5,6,7-tetrahydro-2H-indazole-3-carboximidamide
Overview
Description
2-ethyl-4,5,6,7-tetrahydro-2H-indazole-3-carboximidamide is a useful research compound. Its molecular formula is C10H16N4 and its molecular weight is 192.26 g/mol. The purity is usually 95%.
BenchChem offers high-quality this compound suitable for many research applications. Different packaging options are available to accommodate customers' requirements. Please inquire for more information about this compound including the price, delivery time, and more detailed information at info@benchchem.com.
Mechanism of Action
- Additionally, indazole may modulate other kinases, such as CHK1 , CHK2 , and h-sgk , which play roles in cancer and other diseases .
Target of Action
- interacts with specific molecular targets within cells. Although the exact targets can vary, some key players include enzymes, receptors, and signaling pathways.
Biochemical Pathways
- impacts several pathways:
- By inhibiting COX-2, it suppresses the production of pro-inflammatory mediators. Modulation of kinases like CHK1 and CHK2 affects cell cycle progression and DNA repair. In insects, indazole derivatives target the ecdysone receptor, disrupting insect development .
Biochemical Analysis
Biochemical Properties
2-Ethyl-4,5,6,7-tetrahydro-2H-indazole-3-carboximidamide plays a significant role in various biochemical reactions. It interacts with several enzymes and proteins, influencing their activity. For instance, it has been observed to inhibit cyclo-oxygenase-2 (COX-2), an enzyme involved in the inflammatory response . Additionally, this compound interacts with matrix metalloproteinases (MMPs), which are involved in the breakdown of extracellular matrix components . These interactions suggest that this compound may have potential therapeutic applications in inflammatory and degenerative diseases.
Cellular Effects
The effects of this compound on various cell types and cellular processes are profound. It has been shown to influence cell signaling pathways, particularly those involved in inflammation and cell proliferation . For example, it can inhibit the production of prostaglandin E2 (PGE2) and tumor necrosis factor-alpha (TNF-α), both of which are key mediators of inflammation . Furthermore, this compound affects gene expression by modulating the activity of transcription factors involved in inflammatory responses . These cellular effects highlight its potential as an anti-inflammatory and anticancer agent.
Molecular Mechanism
At the molecular level, this compound exerts its effects through several mechanisms. It binds to the active sites of enzymes such as COX-2, inhibiting their activity and thereby reducing the production of inflammatory mediators . Additionally, it can modulate the activity of transcription factors, leading to changes in gene expression . This compound also interacts with cell surface receptors, influencing signal transduction pathways that regulate cell proliferation and apoptosis . These molecular mechanisms underpin its potential therapeutic effects.
Temporal Effects in Laboratory Settings
In laboratory settings, the effects of this compound have been observed to change over time. The compound is relatively stable under standard laboratory conditions, but it may degrade over extended periods or under specific conditions such as high temperatures or extreme pH . Long-term studies have shown that it can have sustained effects on cellular function, particularly in reducing inflammation and inhibiting cell proliferation . These temporal effects are crucial for understanding its potential therapeutic applications and optimizing its use in clinical settings.
Dosage Effects in Animal Models
The effects of this compound vary with different dosages in animal models. At lower doses, it has been shown to effectively reduce inflammation and inhibit tumor growth without significant adverse effects . At higher doses, it may cause toxicity, including liver and kidney damage . These dosage effects highlight the importance of determining the optimal therapeutic dose to maximize efficacy while minimizing adverse effects.
Metabolic Pathways
This compound is involved in several metabolic pathways. It is metabolized primarily in the liver by cytochrome P450 enzymes, which convert it into various metabolites . These metabolites may have different biological activities and contribute to the overall effects of the compound . Additionally, this compound can influence metabolic flux by modulating the activity of key enzymes involved in metabolic pathways . Understanding these metabolic pathways is essential for predicting its pharmacokinetics and potential drug interactions.
Transport and Distribution
Within cells and tissues, this compound is transported and distributed through various mechanisms. It can interact with transporters and binding proteins that facilitate its uptake and distribution . Once inside the cells, it may localize to specific compartments, such as the cytoplasm or nucleus, where it exerts its effects . The distribution of this compound within tissues can influence its therapeutic efficacy and potential side effects.
Subcellular Localization
The subcellular localization of this compound is critical for its activity and function. It has been observed to localize primarily in the cytoplasm and nucleus, where it can interact with various biomolecules . This localization is facilitated by specific targeting signals and post-translational modifications that direct the compound to these compartments . Understanding its subcellular localization is essential for elucidating its mechanism of action and optimizing its therapeutic potential.
Biological Activity
2-Ethyl-4,5,6,7-tetrahydro-2H-indazole-3-carboximidamide is a nitrogen-containing heterocyclic compound belonging to the indazole family. This compound has garnered attention in medicinal chemistry due to its unique structural features and potential therapeutic applications. The compound's chemical formula is , with a molecular weight of 192.26 g/mol. This article reviews the biological activity of this compound, focusing on its pharmacological properties, synthesis methods, and potential therapeutic applications.
Synthesis Methods
The synthesis of this compound can be achieved through various methods, including:
- Palladium-Catalyzed Reactions : These reactions are efficient for constructing the indazole core.
- Radical Cyclizations : This method allows for the formation of the bicyclic structure characteristic of indazoles.
These synthetic routes emphasize efficiency and yield in producing this compound, which is crucial for further biological evaluations.
Pharmacological Properties
The biological activities of this compound have been explored in several studies:
- Anti-inflammatory Activity : The compound has demonstrated significant anti-inflammatory effects. In vitro studies have indicated that it inhibits cyclooxygenase-2 (COX-2), an enzyme involved in inflammation processes .
- Antimicrobial Activity : Preliminary evaluations suggest that derivatives of indazole compounds exhibit antimicrobial properties. For instance, related compounds have shown effectiveness against protozoan infections and certain fungal strains like Candida albicans and Candida glabrata .
Interaction Studies
Studies focusing on the interaction of this compound with various biological targets have revealed:
- Binding Affinity : The compound's interaction with enzymes and receptors is crucial for understanding its pharmacodynamics. Binding affinity studies indicate potential therapeutic uses in treating conditions associated with inflammation and microbial infections .
Comparative Analysis with Related Compounds
To understand the unique properties of this compound better, a comparison with structurally similar compounds is beneficial:
Compound Name | Structure Characteristics | Unique Aspects |
---|---|---|
4-Aminoindazole | Contains an amino group at position 4 | Known for its role in cancer therapy |
Indazoles with Carboxylic Acids | Variations include different carboxylic acid substituents | Enhanced solubility and bioavailability |
1H-Indazole Derivatives | Different substitutions on the indazole ring | Diverse biological activities |
The unique tetrahydro configuration combined with ethyl and carboximidamide groups may provide distinct biological activities compared to other indazoles.
Case Studies and Research Findings
Recent research has highlighted the potential of indazole derivatives in drug development:
- In Vitro Studies : Compounds derived from tetrahydroindazoles have shown promising results against various protozoa and fungi. For example, some derivatives were found to be more potent than metronidazole against Giardia intestinalis and Entamoeba histolytica .
- Cytotoxicity Assessments : Selected derivatives exhibited low cytotoxicity in human cell lines (HaCaT and HeLa), indicating their safety profile for future therapeutic applications .
- Mechanisms of Action : Further research is needed to elucidate the mechanisms by which these compounds exert their biological effects. Understanding these pathways will be crucial for optimizing their pharmacological profiles .
Properties
IUPAC Name |
2-ethyl-4,5,6,7-tetrahydroindazole-3-carboximidamide | |
---|---|---|
Source | PubChem | |
URL | https://pubchem.ncbi.nlm.nih.gov | |
Description | Data deposited in or computed by PubChem | |
InChI |
InChI=1S/C10H16N4/c1-2-14-9(10(11)12)7-5-3-4-6-8(7)13-14/h2-6H2,1H3,(H3,11,12) | |
Source | PubChem | |
URL | https://pubchem.ncbi.nlm.nih.gov | |
Description | Data deposited in or computed by PubChem | |
InChI Key |
LFDHFDRCQIFRGM-UHFFFAOYSA-N | |
Source | PubChem | |
URL | https://pubchem.ncbi.nlm.nih.gov | |
Description | Data deposited in or computed by PubChem | |
Canonical SMILES |
CCN1C(=C2CCCCC2=N1)C(=N)N | |
Source | PubChem | |
URL | https://pubchem.ncbi.nlm.nih.gov | |
Description | Data deposited in or computed by PubChem | |
Molecular Formula |
C10H16N4 | |
Source | PubChem | |
URL | https://pubchem.ncbi.nlm.nih.gov | |
Description | Data deposited in or computed by PubChem | |
Molecular Weight |
192.26 g/mol | |
Source | PubChem | |
URL | https://pubchem.ncbi.nlm.nih.gov | |
Description | Data deposited in or computed by PubChem | |
Retrosynthesis Analysis
AI-Powered Synthesis Planning: Our tool employs the Template_relevance Pistachio, Template_relevance Bkms_metabolic, Template_relevance Pistachio_ringbreaker, Template_relevance Reaxys, Template_relevance Reaxys_biocatalysis model, leveraging a vast database of chemical reactions to predict feasible synthetic routes.
One-Step Synthesis Focus: Specifically designed for one-step synthesis, it provides concise and direct routes for your target compounds, streamlining the synthesis process.
Accurate Predictions: Utilizing the extensive PISTACHIO, BKMS_METABOLIC, PISTACHIO_RINGBREAKER, REAXYS, REAXYS_BIOCATALYSIS database, our tool offers high-accuracy predictions, reflecting the latest in chemical research and data.
Strategy Settings
Precursor scoring | Relevance Heuristic |
---|---|
Min. plausibility | 0.01 |
Model | Template_relevance |
Template Set | Pistachio/Bkms_metabolic/Pistachio_ringbreaker/Reaxys/Reaxys_biocatalysis |
Top-N result to add to graph | 6 |
Feasible Synthetic Routes
Disclaimer and Information on In-Vitro Research Products
Please be aware that all articles and product information presented on BenchChem are intended solely for informational purposes. The products available for purchase on BenchChem are specifically designed for in-vitro studies, which are conducted outside of living organisms. In-vitro studies, derived from the Latin term "in glass," involve experiments performed in controlled laboratory settings using cells or tissues. It is important to note that these products are not categorized as medicines or drugs, and they have not received approval from the FDA for the prevention, treatment, or cure of any medical condition, ailment, or disease. We must emphasize that any form of bodily introduction of these products into humans or animals is strictly prohibited by law. It is essential to adhere to these guidelines to ensure compliance with legal and ethical standards in research and experimentation.