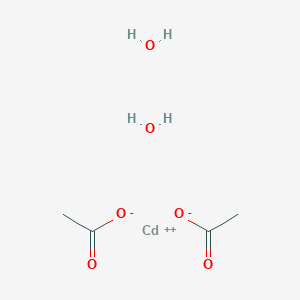
Cadmium acetate dihydrate
- Click on QUICK INQUIRY to receive a quote from our team of experts.
- With the quality product at a COMPETITIVE price, you can focus more on your research.
Overview
Description
Cadmium acetate is a chemical compound with the formula Cd(O₂CCH₃)₂(H₂O)₂. It is available in both anhydrous and dihydrate forms, both of which are white or colorless. The dihydrate form has been verified by X-ray crystallography . Cadmium acetate is primarily used in the synthesis of other cadmium compounds and has applications in various scientific fields.
Preparation Methods
Cadmium acetate can be synthesized through several methods:
- Cadmium oxide reacts with acetic acid and water to form cadmium acetate dihydrate:
Reaction with Acetic Acid: CdO+2CH₃CO₂H+H₂O→Cd(O₂CCH₃)₂(H₂O)₂
Reaction with Acetic Anhydride: Cadmium nitrate reacts with acetic anhydride to produce cadmium acetate.
Industrial production methods often involve these reactions under controlled conditions to ensure high purity and yield.
Chemical Reactions Analysis
Cadmium acetate undergoes various chemical reactions:
Oxidation: Cadmium acetate can be oxidized to form cadmium oxide.
Reduction: It can be reduced to elemental cadmium.
Substitution: Cadmium acetate reacts with trioctylphosphine selenide to form cadmium selenide, a semiconductor material.
Complex Formation: Cadmium acetate forms complexes with ligands such as ammonia, resulting in compounds like [Cd(NH₃)₄]²⁺.
Common reagents used in these reactions include acetic acid, acetic anhydride, and trioctylphosphine selenide. Major products formed include cadmium oxide, cadmium selenide, and various cadmium complexes.
Scientific Research Applications
Cadmium acetate has several applications in scientific research:
Mechanism of Action
Cadmium acetate exerts its effects primarily through its interaction with cellular proteins and enzymes. Cadmium ions can bind to metallothionein in the liver and kidneys, leading to nephrotoxicity. Cadmium also inhibits DNA repair enzymes, contributing to its carcinogenic effects . The molecular targets include metallothionein and various DNA repair proteins.
Comparison with Similar Compounds
Cadmium acetate can be compared with other cadmium compounds such as:
- Cadmium Chloride (CdCl₂)
- Cadmium Sulfide (CdS)
- Cadmium Oxide (CdO)
- Cadmium Nitrate (Cd(NO₃)₂)
Cadmium acetate is unique in its ability to form complexes with organic ligands and its use as a precursor for semiconductor materials. Unlike cadmium chloride and cadmium nitrate, cadmium acetate is less soluble in water, which can be advantageous in certain applications .
Properties
CAS No. |
5743-04-4 |
---|---|
Molecular Formula |
C2H6CdO3 |
Molecular Weight |
190.48 g/mol |
IUPAC Name |
acetic acid;cadmium;hydrate |
InChI |
InChI=1S/C2H4O2.Cd.H2O/c1-2(3)4;;/h1H3,(H,3,4);;1H2 |
InChI Key |
DJEVLLXYYDXCCO-UHFFFAOYSA-N |
SMILES |
CC(=O)[O-].CC(=O)[O-].O.O.[Cd+2] |
Canonical SMILES |
CC(=O)O.O.[Cd] |
Color/Form |
Colorless crystals Monoclinic, colorless crystals |
density |
2.34 at 68 °F (USCG, 1999) - Denser than water; will sink 2.34 g/cu cm Becomes anhydrous at about 130 °C; density 2.01 g/cu cm; pH of 0.2 molar aq soln 7.1; colorless crystals; freely soluble in water; soluble in alcohol /Cadmium acetate dihydrate/ 2.34 g/cm³ |
melting_point |
255 °C |
physical_description |
Cadmium acetate is an odorless colorless solid. Sinks and mixes with water. (USCG, 1999) Colorless solid; [HSDB] White powder; [MSDSonline] COLOURLESS CRYSTALS WITH CHARACTERISTIC ODOUR. |
Pictograms |
Acute Toxic; Irritant; Health Hazard |
Related CAS |
22429-86-3 (trihydrate) 5743-04-4 (dihydrate) 543-90-8 (Parent) |
solubility |
Soluble in water, ethanol Solubility in water: very good |
Synonyms |
cadmium acetate cadmium acetate, dihydrate cadmium acetate, trihydrate |
Origin of Product |
United States |
Retrosynthesis Analysis
AI-Powered Synthesis Planning: Our tool employs the Template_relevance Pistachio, Template_relevance Bkms_metabolic, Template_relevance Pistachio_ringbreaker, Template_relevance Reaxys, Template_relevance Reaxys_biocatalysis model, leveraging a vast database of chemical reactions to predict feasible synthetic routes.
One-Step Synthesis Focus: Specifically designed for one-step synthesis, it provides concise and direct routes for your target compounds, streamlining the synthesis process.
Accurate Predictions: Utilizing the extensive PISTACHIO, BKMS_METABOLIC, PISTACHIO_RINGBREAKER, REAXYS, REAXYS_BIOCATALYSIS database, our tool offers high-accuracy predictions, reflecting the latest in chemical research and data.
Strategy Settings
Precursor scoring | Relevance Heuristic |
---|---|
Min. plausibility | 0.01 |
Model | Template_relevance |
Template Set | Pistachio/Bkms_metabolic/Pistachio_ringbreaker/Reaxys/Reaxys_biocatalysis |
Top-N result to add to graph | 6 |
Feasible Synthetic Routes
Q1: What is the molecular formula and weight of cadmium acetate dihydrate?
A1: The molecular formula of this compound is Cd(CH3COO)2·2H2O, and its molecular weight is 266.52 g/mol.
Q2: What spectroscopic techniques are commonly used to characterize this compound?
A2: Researchers frequently employ techniques like X-ray diffraction (XRD) [, , , , , , , , ], Fourier transform infrared (FTIR) spectroscopy [, , , , , ], Raman spectroscopy [, ], nuclear magnetic resonance (NMR) spectroscopy [, , ], and X-ray photoelectron spectroscopy (XPS) [] to characterize this compound and its derivatives.
Q3: What is the thermal stability of this compound?
A3: Thermogravimetric analysis (TGA) reveals that this compound remains stable up to approximately 650 °C. [, ]
Q4: How does the choice of solvent affect the synthesis of this compound-based nanomaterials?
A4: Solvents play a crucial role in controlling the size and morphology of nanomaterials. For instance, using a mixture of ethylene glycol and water as a solvent during the solvothermal synthesis of cadmium selenide nanoparticles enables precise size control. []
Q5: How is this compound used in the synthesis of nanomaterials?
A5: this compound serves as a common cadmium source in various nanomaterial syntheses. For example, it's used to create cadmium sulfide (CdS) nanoparticles [, , , , , , , , ] and cadmium selenide (CdSe) quantum dots [, , , , , , ] with controlled size and optical properties.
Q6: How is computational chemistry employed in studying this compound?
A6: Researchers utilize computational methods, including density functional theory (DFT) calculations, to predict properties like chemical shifts in cadmium complexes, including this compound. [] These calculations provide insights into the electronic structure and reactivity of the compound.
Q7: What are the known toxicological effects of cadmium?
A7: Cadmium exposure can have detrimental effects on various organs. Studies indicate that cadmium can negatively impact liver function by affecting microsomal hemoproteins and heme oxygenase. [] Additionally, cadmium exposure raises concerns about potential toxicity to the reproductive system. []
Q8: What types of nanomaterials can be synthesized using this compound?
A8: this compound is a versatile precursor for synthesizing a variety of nanomaterials, including:
- Quantum dots: Cadmium selenide (CdSe) quantum dots with tunable bandgaps and photoluminescence properties can be synthesized using this compound. [, , , , , ]
- Nanoparticles: Cadmium sulfide (CdS) nanoparticles with controlled size and morphology are produced using this compound as a cadmium source. [, , , , , , , , ]
- Nanorods: Cadmium sulfide (CdS) nanorods with a preferential growth direction can be synthesized through pyrolysis using this compound and sulfur powder. []
Disclaimer and Information on In-Vitro Research Products
Please be aware that all articles and product information presented on BenchChem are intended solely for informational purposes. The products available for purchase on BenchChem are specifically designed for in-vitro studies, which are conducted outside of living organisms. In-vitro studies, derived from the Latin term "in glass," involve experiments performed in controlled laboratory settings using cells or tissues. It is important to note that these products are not categorized as medicines or drugs, and they have not received approval from the FDA for the prevention, treatment, or cure of any medical condition, ailment, or disease. We must emphasize that any form of bodily introduction of these products into humans or animals is strictly prohibited by law. It is essential to adhere to these guidelines to ensure compliance with legal and ethical standards in research and experimentation.