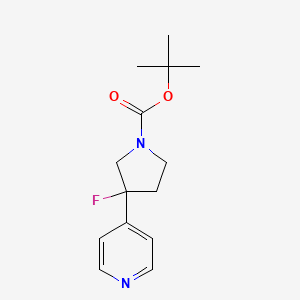
Tert-butyl 3-fluoro-3-(pyridin-4-yl)pyrrolidine-1-carboxylate
Overview
Description
Synthesis Analysis
The synthesis of similar compounds has been reported in the literature . For instance, a new series of 4-(1-(tert-butyl)-3-phenyl-1H-pyrazol-4-yl) pyridine possessing terminal ethyl or propyl sulfonamides was designed and synthesized . The cytotoxic effect of the final compounds was measured by applying MTT assay in LPS-Induced RAW264.7 macrophage cells .Molecular Structure Analysis
The molecular structure of similar compounds has been reported. For example, the molecular formula of “tert-Butyl (3S,4R)-4-fluoro-3-hydroxypiperidine-1-carboxylate” is CHFNO with an average mass of 219.253 Da and a monoisotopic mass of 219.127075 Da .Physical and Chemical Properties Analysis
The physical and chemical properties of similar compounds have been reported. For instance, “tert-butyl (3R)-3-fluoro-3-(hydroxymethyl)pyrrolidine-1-carboxylate” has a molecular formula of CHFNO .Scientific Research Applications
Subheading Metabolism of Novel Dipeptidyl Peptidase-4 Inhibitor
The study by Yoo et al. (2008) focused on the in vitro metabolism of a novel dipeptidyl peptidase-4 inhibitor, involving tert-butyl moiety. The research explored the enzymatic C-demethylation of this compound in rat liver microsomes, highlighting the major metabolic reactions like hydroxylation and carbonyl reduction. It particularly noted the formation of a C-demethylated metabolite through nonenzymatic decarboxylation, influenced by the resonance stabilization by an attached oxadiazole ring (Yoo et al., 2008).
Synthetic Chemistry and Asymmetric Synthesis
Subheading Enantioselective Synthesis of Substituted Pyrrolidines
Chung et al. (2005) described an enantioselective synthesis of N-tert-butyl disubstituted pyrrolidines using a nitrile anion cyclization strategy. This method achieved high yield and enantiomeric excess, demonstrating its practicality for synthesizing chiral pyrrolidines with potential applications in various fields (Chung et al., 2005).
Subheading Crystal Structure Analysis
Naveen et al. (2007) undertook the synthesis and characterization of tert-butyl 2-((phenylthio)carbonyl)pyrrolidine-1-carboxylate, providing valuable insights into its crystal structure through X-ray diffraction studies. The findings contribute to a deeper understanding of the molecular conformation and interactions within the structure, emphasizing the significance of hydrogen bonds in stabilizing the molecular arrangement (Naveen et al., 2007).
Subheading Determining Absolute Configuration
Procopiou et al. (2016) focused on determining the absolute configuration of a related fluoropyrrolidine compound and confirming its stereochemistry through vibrational circular dichroism and chemical synthesis. The research underscores the importance of accurate stereochemical characterization in the development and application of such compounds (Procopiou et al., 2016).
Mechanism of Action
Target of Action
Compounds with similar structures, such as indole derivatives, have been found to bind with high affinity to multiple receptors , suggesting that this compound may also interact with various biological targets.
Mode of Action
For instance, indole derivatives are known to interact with their targets and cause changes that lead to various biological activities .
Biochemical Pathways
Related compounds, such as indole derivatives, are known to affect various biological activities, including antiviral, anti-inflammatory, anticancer, anti-hiv, antioxidant, antimicrobial, antitubercular, antidiabetic, antimalarial, and anticholinesterase activities . This suggests that Tert-butyl 3-fluoro-3-(pyridin-4-yl)pyrrolidine-1-carboxylate may also influence a range of biochemical pathways.
Pharmacokinetics
The introduction of heteroatomic fragments in drug molecules, as seen in this compound, is a common strategy for modifying physicochemical parameters and obtaining the best adme/tox results for drug candidates .
Result of Action
Based on the known effects of similar compounds, it can be inferred that this compound may have a broad spectrum of biological activities .
Properties
IUPAC Name |
tert-butyl 3-fluoro-3-pyridin-4-ylpyrrolidine-1-carboxylate | |
---|---|---|
Source | PubChem | |
URL | https://pubchem.ncbi.nlm.nih.gov | |
Description | Data deposited in or computed by PubChem | |
InChI |
InChI=1S/C14H19FN2O2/c1-13(2,3)19-12(18)17-9-6-14(15,10-17)11-4-7-16-8-5-11/h4-5,7-8H,6,9-10H2,1-3H3 | |
Source | PubChem | |
URL | https://pubchem.ncbi.nlm.nih.gov | |
Description | Data deposited in or computed by PubChem | |
InChI Key |
RIYZBYSYZRMHAR-UHFFFAOYSA-N | |
Source | PubChem | |
URL | https://pubchem.ncbi.nlm.nih.gov | |
Description | Data deposited in or computed by PubChem | |
Canonical SMILES |
CC(C)(C)OC(=O)N1CCC(C1)(C2=CC=NC=C2)F | |
Source | PubChem | |
URL | https://pubchem.ncbi.nlm.nih.gov | |
Description | Data deposited in or computed by PubChem | |
Molecular Formula |
C14H19FN2O2 | |
Source | PubChem | |
URL | https://pubchem.ncbi.nlm.nih.gov | |
Description | Data deposited in or computed by PubChem | |
Molecular Weight |
266.31 g/mol | |
Source | PubChem | |
URL | https://pubchem.ncbi.nlm.nih.gov | |
Description | Data deposited in or computed by PubChem | |
Retrosynthesis Analysis
AI-Powered Synthesis Planning: Our tool employs the Template_relevance Pistachio, Template_relevance Bkms_metabolic, Template_relevance Pistachio_ringbreaker, Template_relevance Reaxys, Template_relevance Reaxys_biocatalysis model, leveraging a vast database of chemical reactions to predict feasible synthetic routes.
One-Step Synthesis Focus: Specifically designed for one-step synthesis, it provides concise and direct routes for your target compounds, streamlining the synthesis process.
Accurate Predictions: Utilizing the extensive PISTACHIO, BKMS_METABOLIC, PISTACHIO_RINGBREAKER, REAXYS, REAXYS_BIOCATALYSIS database, our tool offers high-accuracy predictions, reflecting the latest in chemical research and data.
Strategy Settings
Precursor scoring | Relevance Heuristic |
---|---|
Min. plausibility | 0.01 |
Model | Template_relevance |
Template Set | Pistachio/Bkms_metabolic/Pistachio_ringbreaker/Reaxys/Reaxys_biocatalysis |
Top-N result to add to graph | 6 |
Feasible Synthetic Routes
Disclaimer and Information on In-Vitro Research Products
Please be aware that all articles and product information presented on BenchChem are intended solely for informational purposes. The products available for purchase on BenchChem are specifically designed for in-vitro studies, which are conducted outside of living organisms. In-vitro studies, derived from the Latin term "in glass," involve experiments performed in controlled laboratory settings using cells or tissues. It is important to note that these products are not categorized as medicines or drugs, and they have not received approval from the FDA for the prevention, treatment, or cure of any medical condition, ailment, or disease. We must emphasize that any form of bodily introduction of these products into humans or animals is strictly prohibited by law. It is essential to adhere to these guidelines to ensure compliance with legal and ethical standards in research and experimentation.