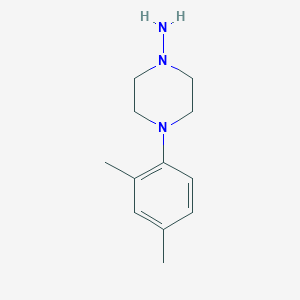
4-(2,4-Dimethylphenyl)piperazin-1-amine
Overview
Description
Synthesis Analysis
Piperazine derivatives can be synthesized through various methods, including cyclization of 1,2-diamine derivatives with sulfonium salts, the Ugi reaction, ring opening of aziridines under the action of N-nucleophiles, intermolecular cycloaddition of alkynes bearing an amino group, parallel solid-phase synthesis, and photocatalytic synthesis . For instance, the ring formation reaction between (S,S)-N,N’-bisnosyl diamine and diphenylvinylsulfonium triflate in the presence of DBU leads to protected piperazines .Chemical Reactions Analysis
The chemical reactions involving piperazine derivatives are diverse. For instance, the reaction of protected 1,2-diamines with 2-bromoethyldiphenylsulfonium triflate under basic conditions leads to the synthesis of 2-substituted chiral piperazines .Scientific Research Applications
Piperazine Derivatives in Therapeutic Development
Piperazine derivatives, including 4-(2,4-Dimethylphenyl)piperazin-1-amine, have garnered attention due to their significant presence in a variety of drugs with diverse therapeutic uses. These applications span across antipsychotic, antihistamine, antianginal, antidepressant, anticancer, antiviral, cardioprotective, anti-inflammatory, and imaging agents, demonstrating the versatile medicinal potential of piperazine compounds. The modification of the substitution pattern on the piperazine nucleus can distinctly alter the medicinal properties of the resultant molecules, indicating the critical role of structural design in the development of new therapeutics. This versatility suggests a broad potential for piperazine-based molecules in drug discovery and development, encouraging further therapeutic investigations on this motif for a wide array of diseases (Rathi et al., 2016).
Anti-mycobacterial Activity and SAR of Piperazine Analogues
Piperazine and its analogues have shown significant promise in combating Mycobacterium tuberculosis (MTB), including multidrug-resistant (MDR) and extremely drug-resistant (XDR) strains. The review on anti-mycobacterial compounds spanning five decades highlights the importance of piperazine as a key building block in the design of potent anti-TB molecules. This body of work aids medicinal chemists in understanding the design rationale and structure-activity relationship (SAR) of these compounds, facilitating the development of safer, selective, and cost-effective anti-mycobacterial agents. The inclusion of piperazine in drug frameworks underscores its potential in addressing the pressing need for new treatments against tuberculosis (Girase et al., 2020).
Mechanism of Action
Target of Action
It is known that some piperazine derivatives have significant pharmacophoric activities . They have been tested for antibacterial and antifungal activities against various organisms such as Staphylococcus aureus, Streptomyces epidermidis, Pseudomonas aeruginosa, Escherichia coli, Candida albicans, Aspergillus niger, Aspergillus flavus, and Aspergillus fumigatus .
Mode of Action
It is known that piperazine derivatives can interfere with the natural life cycle of bacteria, thus creating evolutionary pressure on them .
Biochemical Pathways
It is known that piperazine derivatives can affect a wide range of biological and pharmaceutical activities .
Result of Action
Many synthesized compounds of the piperazine family have shown significant antimicrobial and antifungal properties .
Biochemical Analysis
Biochemical Properties
4-(2,4-Dimethylphenyl)piperazin-1-amine plays a significant role in biochemical reactions by interacting with various enzymes, proteins, and other biomolecules. It has been observed to interact with acetylcholinesterase, an enzyme responsible for hydrolyzing acetylcholine in the brain. The interaction between this compound and acetylcholinesterase results in the inhibition of the enzyme’s activity, which can have implications for cognitive functions . Additionally, this compound has shown interactions with other proteins involved in neurotransmission, potentially affecting synaptic signaling and plasticity.
Cellular Effects
The effects of this compound on various types of cells and cellular processes are profound. In neuronal cells, it has been shown to influence cell signaling pathways, particularly those related to neurotransmitter release and uptake. This compound can modulate gene expression, leading to changes in the production of proteins involved in synaptic function and plasticity. Furthermore, this compound affects cellular metabolism by altering the activity of enzymes involved in energy production and utilization .
Molecular Mechanism
At the molecular level, this compound exerts its effects through several mechanisms. It binds to acetylcholinesterase, inhibiting its activity and preventing the breakdown of acetylcholine. This inhibition leads to an accumulation of acetylcholine in the synaptic cleft, enhancing cholinergic signaling. Additionally, this compound can modulate the activity of other enzymes and proteins involved in neurotransmission, further influencing synaptic function and plasticity . Changes in gene expression induced by this compound also contribute to its molecular effects, as it can upregulate or downregulate the production of specific proteins.
Temporal Effects in Laboratory Settings
In laboratory settings, the effects of this compound have been observed to change over time. The compound exhibits stability under controlled conditions, but its degradation can occur over extended periods. Long-term exposure to this compound has been shown to result in sustained changes in cellular function, including alterations in gene expression and enzyme activity. These temporal effects highlight the importance of considering the duration of exposure when studying the biochemical properties of this compound .
Dosage Effects in Animal Models
The effects of this compound vary with different dosages in animal models. At low doses, the compound has been observed to enhance cognitive functions and improve memory retention. At higher doses, it can lead to toxic effects, including neurotoxicity and impaired motor function. Threshold effects have been identified, indicating that there is a specific dosage range within which the compound exerts its beneficial effects without causing adverse reactions .
Metabolic Pathways
This compound is involved in several metabolic pathways, interacting with enzymes and cofactors that regulate its metabolism. The compound can influence metabolic flux by altering the activity of key enzymes involved in energy production and utilization. Additionally, it can affect metabolite levels, leading to changes in the concentrations of specific molecules within cells .
Transport and Distribution
Within cells and tissues, this compound is transported and distributed through interactions with transporters and binding proteins. These interactions facilitate the localization and accumulation of the compound in specific cellular compartments. The distribution of this compound can influence its activity and function, as its presence in different cellular regions can affect its interactions with biomolecules .
Subcellular Localization
The subcellular localization of this compound is critical for its activity and function. The compound can be directed to specific compartments or organelles through targeting signals and post-translational modifications. Its localization within cells can influence its interactions with enzymes, proteins, and other biomolecules, ultimately affecting its biochemical properties and cellular effects .
properties
IUPAC Name |
4-(2,4-dimethylphenyl)piperazin-1-amine | |
---|---|---|
Source | PubChem | |
URL | https://pubchem.ncbi.nlm.nih.gov | |
Description | Data deposited in or computed by PubChem | |
InChI |
InChI=1S/C12H19N3/c1-10-3-4-12(11(2)9-10)14-5-7-15(13)8-6-14/h3-4,9H,5-8,13H2,1-2H3 | |
Source | PubChem | |
URL | https://pubchem.ncbi.nlm.nih.gov | |
Description | Data deposited in or computed by PubChem | |
InChI Key |
VENRVVIBVZXKFG-UHFFFAOYSA-N | |
Source | PubChem | |
URL | https://pubchem.ncbi.nlm.nih.gov | |
Description | Data deposited in or computed by PubChem | |
Canonical SMILES |
CC1=CC(=C(C=C1)N2CCN(CC2)N)C | |
Source | PubChem | |
URL | https://pubchem.ncbi.nlm.nih.gov | |
Description | Data deposited in or computed by PubChem | |
Molecular Formula |
C12H19N3 | |
Source | PubChem | |
URL | https://pubchem.ncbi.nlm.nih.gov | |
Description | Data deposited in or computed by PubChem | |
Molecular Weight |
205.30 g/mol | |
Source | PubChem | |
URL | https://pubchem.ncbi.nlm.nih.gov | |
Description | Data deposited in or computed by PubChem | |
Retrosynthesis Analysis
AI-Powered Synthesis Planning: Our tool employs the Template_relevance Pistachio, Template_relevance Bkms_metabolic, Template_relevance Pistachio_ringbreaker, Template_relevance Reaxys, Template_relevance Reaxys_biocatalysis model, leveraging a vast database of chemical reactions to predict feasible synthetic routes.
One-Step Synthesis Focus: Specifically designed for one-step synthesis, it provides concise and direct routes for your target compounds, streamlining the synthesis process.
Accurate Predictions: Utilizing the extensive PISTACHIO, BKMS_METABOLIC, PISTACHIO_RINGBREAKER, REAXYS, REAXYS_BIOCATALYSIS database, our tool offers high-accuracy predictions, reflecting the latest in chemical research and data.
Strategy Settings
Precursor scoring | Relevance Heuristic |
---|---|
Min. plausibility | 0.01 |
Model | Template_relevance |
Template Set | Pistachio/Bkms_metabolic/Pistachio_ringbreaker/Reaxys/Reaxys_biocatalysis |
Top-N result to add to graph | 6 |
Feasible Synthetic Routes
Disclaimer and Information on In-Vitro Research Products
Please be aware that all articles and product information presented on BenchChem are intended solely for informational purposes. The products available for purchase on BenchChem are specifically designed for in-vitro studies, which are conducted outside of living organisms. In-vitro studies, derived from the Latin term "in glass," involve experiments performed in controlled laboratory settings using cells or tissues. It is important to note that these products are not categorized as medicines or drugs, and they have not received approval from the FDA for the prevention, treatment, or cure of any medical condition, ailment, or disease. We must emphasize that any form of bodily introduction of these products into humans or animals is strictly prohibited by law. It is essential to adhere to these guidelines to ensure compliance with legal and ethical standards in research and experimentation.