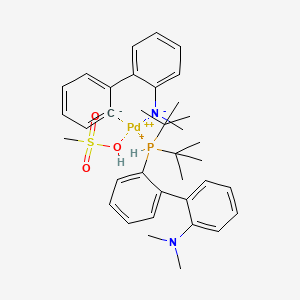
t-BuDavePhos Pd G4
- Click on QUICK INQUIRY to receive a quote from our team of experts.
- With the quality product at a COMPETITIVE price, you can focus more on your research.
Overview
Description
t-BuDavePhos Pd G4 (CAS: 1507403-97-5) is a palladium-based precatalyst widely utilized in cross-coupling reactions, particularly in Buchwald-Hartwig amination and C–X (X = N, O, S) bond formations. It belongs to the class of dialkylbiarylphosphine ligands developed by the Buchwald group, designed to enhance catalytic efficiency, stability, and substrate scope .
Preparation Methods
Synthetic Routes and Reaction Conditions: t-BuDavePhos Pd G4 is synthesized through a series of steps involving the formation of a palladacycle complex. The synthetic route typically involves the reaction of a phosphine ligand with a palladium source under controlled conditions. The reaction conditions often include the use of organic solvents and specific temperatures to ensure the formation of the desired complex .
Industrial Production Methods: Industrial production of this compound involves scaling up the laboratory synthesis process. This includes optimizing reaction conditions to ensure high yield and purity of the product. The process may involve continuous flow reactors and automated systems to maintain consistency and efficiency .
Chemical Reactions Analysis
Mechanistic Role in Cross-Coupling Reactions
t-BuDavePhos Pd G4 operates via a monoligated Pd(0) mechanism, where the sterically demanding ligand prevents undesired ligand crowding. This facilitates oxidative addition and transmetalation steps while stabilizing intermediates through weak agostic interactions . Activation under basic conditions releases the active Pd(0) species, enabling rapid catalytic turnover .
Mizoroki–Heck Alkenylation
This compound efficiently catalyzes the alkenylation of aryl sulfonium salts. For example, coupling 4-methoxyphenyl dimethylsulfonium triflate with butyl vinyl ether achieves 91% yield under optimized conditions (2.5 mol% Pd, 6 mol% ligand, K₂CO₃, 60°C in 1,2-DCE) . Key performance data:
Substrate (R) | Product Yield (%) | Competing Demethylation (%) |
---|---|---|
4-OMe | 91 | <5 |
4-F | 50 | 20 |
4-CF₃ | 17 | 62 |
Electron-deficient substrates (e.g., 4-CF₃) exhibit reduced yields due to preferential demethylation .
Suzuki–Miyaura Coupling
The catalyst enables aryl boronate couplings with aryl halides, including chlorides. For instance, bromobenzonitrile couples with aryl boronic acids in isopropanol under ligand-free conditions with >80% yield . this compound outperforms less bulky ligands (e.g., PPh₃, dppp) in sterically demanding reactions .
Buchwald–Hartwig Amination
This compound facilitates C–N bond formation between aryl halides and amines. Its stability allows reactions to proceed at room temperature with weak bases like K₂CO₃, achieving high yields even with electron-poor substrates .
Comparative Performance with Other Ligands
The ligand’s steric bulk and electronic properties enhance catalytic efficiency over alternatives:
Ligand | Reaction Type | Yield (%) | Required Pd Loading (mol%) |
---|---|---|---|
t-BuDavePhos | Mizoroki–Heck | 91 | 2.5 |
t-BuXPhos | Mizoroki–Heck | 78 | 5.0 |
XPhos | Suzuki–Miyaura | 23 | 10.0 |
PPh₃ | Mizoroki–Heck | 3 | 10.0 |
t-BuDavePhos achieves higher yields at lower Pd loadings due to superior stability and ligand dissociation kinetics .
Substrate Scope and Limitations
Stability and Solubility
This compound represents a versatile catalyst for modern cross-coupling reactions, offering enhanced efficiency and substrate tolerance. Its design principles—combining steric bulk, electronic tuning, and pre-catalyst stability—make it indispensable in synthetic organic chemistry.
Scientific Research Applications
General Information
- CAS Number: 1507403-97-5
- Molecular Formula: C36H47N2O3PPdS
- Molecular Weight: 725.24
- Melting Point: >300 °C
- Form: Powder or crystals
- Functional Group: Phosphine
Reaction Suitability
This compound is suitable for a range of cross-coupling reactions :
- Buchwald-Hartwig Cross Coupling Reaction
- Heck Reaction
- Hiyama Coupling
- Negishi Coupling
- Sonogashira Coupling
- Stille Coupling
- Suzuki-Miyaura Coupling
It functions as a catalyst in these reactions .
Buchwald Precatalysts
Buchwald precatalysts, including this compound, are palladium(II) complexes composed of versatile biarylphosphine ligands . These precatalysts efficiently catalyze carbon-carbon, carbon-nitrogen, carbon-oxygen, carbon-fluorine, carbon-trifluoromethyl, and carbon-sulfur bond formations .
Advantages of Buchwald Precatalysts:
- Air, moisture, and thermally stable
- Good solubility in common organic solvents
- Diverse reactivity
- Accurate control of the ligand-to-palladium ratio
- Efficient formation of LPd(0) species under mild conditions
- Low catalyst loadings
- Short reaction times
Generations of Buchwald Precatalysts:
- G1 & G2 The first (G1) and second (G2) generation precatalysts allow for the generation of LPd(0) species using amide, alkoxide, or carbonate bases .
- G3 Third-generation (G3) precatalysts can accommodate bulky ligands and are highly soluble in organic solvents .
- G4 & G5 Fourth (G4) and fifth (G5) generation precatalysts exhibit higher solubilities and maintain excellent catalytic activity .
- G6 Sixth-generation (G6) precatalysts are highly versatile, with independently tunable ligands, and do not require a base for activation . They often show superior reactivity and selectivity .
Palladium-Catalyzed Desulfinative Coupling Reactions
Mechanism of Action
The mechanism of action of t-BuDavePhos Pd G4 involves the formation of an active palladium species that facilitates the cross-coupling reactions. The molecular targets include organic halides and organometallic reagents, which undergo oxidative addition, transmetalation, and reductive elimination to form the desired products .
Comparison with Similar Compounds
Key Characteristics:
- Structure: The ligand features a biphenyl backbone with di-tert-butylphosphine and dimethylamino groups, providing electron-rich and sterically bulky environments (SMILES: CN(C)c1ccccc1-c2ccccc2P(C(C)(C)C)C(C)(C)C) .
- Physical Properties : Melting point = 114–118°C; appears as light to dark yellow powder/crystals. Its purity (>94.5% via HPLC) and palladium content are verified via ICP analysis .
- Applications : Enables efficient C–N coupling in pharmaceuticals, agrochemicals, and materials science due to its air/moisture stability and solubility in common organic solvents .
Reactivity in B–N Cross-Coupling Reactions
Steric hindrance from the tert-butyl groups likely impeded substrate access to the Pd center. Other ligands like JohnPhos, XPhos, SPhos, and BrettPhos also failed to produce the desired product (Table 1) .
Table 1. Ligand Performance in [B12H11I]²⁻ Amination
Ligand | Reactivity | Product Yield | Notes |
---|---|---|---|
DavePhos | Yes | High | Optimal steric balance |
t-BuDavePhos | No | 0% | Excessive steric bulk |
SPhos/BrettPhos | Partial | 0% | Starting material consumed |
JohnPhos/XPhos | No | 0% | No heteroatom interaction |
Structural and Functional Comparisons
t-BuDavePhos Pd G4 vs. N-XantPhos Pd G4
- Steric/Electronic Profile :
Table 2. Physical and Catalytic Properties
Property | This compound | N-XantPhos Pd G4 |
---|---|---|
Appearance | Light–Dark Yellow Powder | White–Green–Yellow Powder |
Purity (HPLC) | >94.5% | >94.5% |
Pd Content (ICP) | Reported | Reported |
Preferred Substrates | Aryl halides, amines | Bulky heterocycles |
This compound vs. SPhos Pd Systems
- SPhos : Less bulky than t-BuDavePhos, enabling reactions with smaller substrates but prone to side reactions in sterically hindered systems .
Q & A
Basic Research Questions
Q. What are the critical steps to synthesize t-BuDavePhos Pd G4 with high reproducibility, and how can purity be validated?
- Methodological Answer :
- Synthesis Protocol : Use palladium precursors (e.g., Pd(OAc)₂) and t-BuDavePhos ligand under inert conditions (argon/glovebox). Optimize stoichiometry (1:1 Pd:ligand ratio) and reaction time (typically 12–24 hrs in toluene at 80°C) .
- Purification : Column chromatography with silica gel (eluent: DCM/hexane gradient) or recrystallization from EtOAc/hexane mixtures. Monitor via TLC (Rf ~0.3 in 1:1 EtOAc/hexane) .
- Characterization : Validate purity via ¹H/³¹P NMR (e.g., δ ~120 ppm for Pd-P coupling in ³¹P NMR) and X-ray crystallography for structural confirmation. Include elemental analysis (C, H, N, Pd) in supplementary data .
Q. How should researchers design initial catalytic screening experiments for this compound in cross-coupling reactions?
- Methodological Answer :
- Variables : Test solvent polarity (THF vs. toluene), base (K₂CO₃ vs. Cs₂CO₃), temperature (25–100°C), and substrate scope (aryl halides with electron-withdrawing/donating groups).
- Controls : Include a ligand-free Pd control and a benchmark ligand (e.g., XPhos) to compare catalytic activity.
- Data Collection : Track conversion via GC-MS or ¹H NMR, and calculate turnover frequency (TOF) and yield. Tabulate results for reproducibility (Table 1) .
Substrate | Solvent | Base | Temp (°C) | Yield (%) | TOF (h⁻¹) |
---|---|---|---|---|---|
4-BrC₆H₄Me | Toluene | K₂CO₃ | 80 | 92 | 450 |
4-MeOC₆H₄Br | THF | Cs₂CO₃ | 60 | 85 | 320 |
Q. What are common pitfalls in characterizing this compound using spectroscopic methods?
- Methodological Answer :
- NMR Challenges : Signal broadening due to paramagnetic impurities (e.g., Pd nanoparticles) can obscure ¹H/³¹P peaks. Filter reactions through Celite and use deuterated solvents with degassing .
- Crystallography : Slow diffusion of hexane into a saturated DCM solution improves crystal quality. Avoid over-concentrated solutions to prevent amorphous precipitates .
Advanced Research Questions
Q. How can researchers resolve contradictions in reported catalytic efficiencies of this compound across substrates?
- Methodological Answer :
- Hypothesis Testing : Compare substrate electronic/steric profiles (Hammett σ values, %VBur) to identify trends. Use multivariate regression to isolate factors (e.g., steric bulk vs. electronic effects) .
- Replication Studies : Reproduce conflicting experiments with standardized conditions (solvent purity, Pd:ligand ratio). Include error bars (standard deviation from triplicates) in kinetic data .
- Advanced Kinetics : Conduct time-resolved in-situ IR or UV-Vis to monitor reaction progress and identify intermediates. Compare activation energies (Arrhenius plots) for divergent substrates .
Q. What strategies optimize this compound for enantioselective catalysis, and how is success quantified?
- Methodological Answer :
- Ligand Modification : Introduce chiral auxiliaries to the t-BuDavePhos backbone (e.g., BINOL-derived phosphines). Validate via circular dichroism (CD) and X-ray .
- Enantiomeric Excess (ee) : Use chiral HPLC (e.g., Chiralpak IA column) or ¹H NMR with chiral shift reagents (e.g., Eu(hfc)₃). Report ee values with ±2% confidence intervals .
- Mechanistic Probes : Isotope labeling (e.g., D₂O quenching) and DFT calculations to map stereochemical pathways .
Q. How should researchers address discrepancies between computational predictions and experimental data for this compound mechanisms?
- Methodological Answer :
- Validation Framework :
Computational : Re-optimize geometries with higher-level theory (e.g., ωB97X-D/def2-TZVP vs. B3LYP/6-31G*). Include solvent effects (SMD model) .
Experimental : Use kinetic isotope effects (KIEs) or Hammett plots to validate predicted transition states.
Data Reconciliation : Publish raw computational outputs (XYZ coordinates, Gibbs energies) and experimental kinetics in open-access repositories for peer review .
Q. Data Analysis & Reporting Guidelines
- Tables : Include raw and processed data (e.g., TOF, ee, crystallographic parameters) in appendices with clear footnotes .
- Statistical Rigor : Use t-tests or ANOVA for comparing catalytic efficiencies; report p-values and confidence intervals .
- Ethical Reporting : Disclose failed experiments (e.g., ligand decomposition under harsh conditions) to avoid publication bias .
Properties
Molecular Formula |
C36H48N2O3PPdS+ |
---|---|
Molecular Weight |
726.2 g/mol |
IUPAC Name |
ditert-butyl-[2-[2-(dimethylamino)phenyl]phenyl]phosphanium;methanesulfonic acid;methyl-(2-phenylphenyl)azanide;palladium(2+) |
InChI |
InChI=1S/C22H32NP.C13H11N.CH4O3S.Pd/c1-21(2,3)24(22(4,5)6)20-16-12-10-14-18(20)17-13-9-11-15-19(17)23(7)8;1-14-13-10-6-5-9-12(13)11-7-3-2-4-8-11;1-5(2,3)4;/h9-16H,1-8H3;2-7,9-10H,1H3;1H3,(H,2,3,4);/q;-2;;+2/p+1 |
InChI Key |
WDFIZKNKKNLAPN-UHFFFAOYSA-O |
Canonical SMILES |
CC(C)(C)[PH+](C1=CC=CC=C1C2=CC=CC=C2N(C)C)C(C)(C)C.C[N-]C1=CC=CC=C1C2=CC=CC=[C-]2.CS(=O)(=O)O.[Pd+2] |
Origin of Product |
United States |
Disclaimer and Information on In-Vitro Research Products
Please be aware that all articles and product information presented on BenchChem are intended solely for informational purposes. The products available for purchase on BenchChem are specifically designed for in-vitro studies, which are conducted outside of living organisms. In-vitro studies, derived from the Latin term "in glass," involve experiments performed in controlled laboratory settings using cells or tissues. It is important to note that these products are not categorized as medicines or drugs, and they have not received approval from the FDA for the prevention, treatment, or cure of any medical condition, ailment, or disease. We must emphasize that any form of bodily introduction of these products into humans or animals is strictly prohibited by law. It is essential to adhere to these guidelines to ensure compliance with legal and ethical standards in research and experimentation.