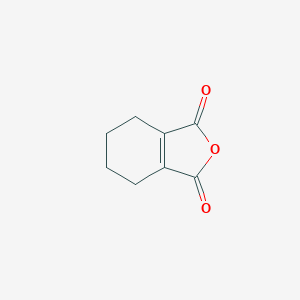
3,4,5,6-Tetrahydrophthalic anhydride
Overview
Description
3,4,5,6-Tetrahydrophthalic anhydride (THPA) is a versatile organic compound that has found a wide range of applications in the fields of organic synthesis, scientific research, and laboratory experiments. It is a white-colored crystalline solid that is formed when phthalic anhydride is heated in the presence of an acid catalyst. THPA is a key intermediate for the synthesis of various organic compounds, and it is also used as a crosslinking agent in polymers and resins. In addition, THPA has been studied extensively for its biochemical and physiological effects, as well as its potential therapeutic applications.
Scientific Research Applications
Crystal Structure Analysis :
- The crystal structure of THPA was analyzed, revealing that it crystallizes in a specific space group with two symmetry-independent molecules per asymmetric unit. The structure evidences considerable distortion of the partially hydrogenated six-membered ring and highlights nonbonded interactions of the dipole–dipole type (Fredj et al., 2004).
Modelling of Crystal Structure :
- Lattice energy calculations and molecular modelling were used to understand the crystal structures of THPA. This study supports the idea that molecules distort from their ideal geometries to improve packing in crystals (Fredj & Day, 2015).
Application in Polyimides :
- THPA was reevaluated as an end cap for improving the oxidation resistance in addition polyimides. This study revealed a balance between cross-linking and aromatization, which contributes to the improved thermooxidative stability of THPA end-capped polyimides (Meador et al., 2004).
Influence of Metal Ions in Chemical Reactions :
- The influence of metal ions on the allylic rearrangement of THPA was studied. The reaction is metal-dependent, leading to the formation of new lamellar coordination polymers with specific metal ions (Pang et al., 2015).
Kinetics of Curing Reaction in Epoxy Systems :
- The curing reaction kinetics of an epoxy system using THPA as an anhydride curing agent was studied. This research provides insights into the effect of the curing agent on the thermomechanical and mechanical properties of the epoxy system (Ding et al., 2017).
Allylic Rearrangement in Water-Rich Reaction :
- A study observed allylic rearrangement when THPA was hydrolyzed in a basic solution, highlighting the chemical behavior of THPA under specific conditions (Pang et al., 2014).
Synthesis and Characterization of Derivatives :
- Research on the synthesis and characterization of new derivatives based on THPA explored its potential in creating novel compounds, which have applications in various industries (Al-Azzawi & Hammud, 2013).
Grafting onto Polyolefins :
- The study on grafting THPA onto polyolefins in solution reveals its potential in modifying polymeric materials, influencing properties like melting temperature and crystallinity (Campos et al., 2004).
Safety and Hazards
Mechanism of Action
Target of Action
3,4,5,6-Tetrahydrophthalic anhydride (THPA) is known to interact with glutamate dehydrogenase . This enzyme plays a crucial role in amino acid metabolism, specifically in the catabolism of glutamate.
Mode of Action
The interaction of THPA with glutamate dehydrogenase results in the progressive loss of enzymatic activity and a concomitant increase in the negative charge of the protein
Pharmacokinetics
Given its molecular weight of 1521473 , it is likely to be absorbed and distributed in the body following administration. The metabolism and excretion of THPA would depend on various factors including the route of administration and the individual’s metabolic rate.
Action Environment
The action, efficacy, and stability of THPA can be influenced by various environmental factors. For instance, the pH of the environment can affect the ionization state of THPA, potentially influencing its interaction with glutamate dehydrogenase Additionally, temperature can impact the rate of enzymatic reactions and thus the degree of enzyme inhibition by THPA
Biochemical Analysis
Biochemical Properties
3,4,5,6-Tetrahydrophthalic anhydride plays a significant role in biochemical reactions, particularly in the modification of enzymes and proteins. One notable interaction is with glutamate dehydrogenase, where this compound modifies the enzyme at pH 8.0, leading to a progressive loss of enzymatic activity and an increase in the negative charge of the protein . This modification highlights the compound’s ability to alter protein structure and function, making it a valuable tool in biochemical studies.
Cellular Effects
The effects of this compound on various cell types and cellular processes are profound. It influences cell function by modifying key enzymes and proteins, which can impact cell signaling pathways, gene expression, and cellular metabolism. For instance, the modification of glutamate dehydrogenase by this compound results in the loss of enzymatic activity, which can affect metabolic pathways and energy production within the cell . This compound’s ability to alter protein function underscores its potential impact on cellular processes.
Molecular Mechanism
At the molecular level, this compound exerts its effects through binding interactions with biomolecules and enzyme inhibition. The compound modifies enzymes such as glutamate dehydrogenase, leading to changes in their activity and function This modification involves the addition of the anhydride group to specific amino acid residues, resulting in altered protein structure and function
Temporal Effects in Laboratory Settings
In laboratory settings, the effects of this compound can change over time due to its stability and degradation. The compound’s stability is influenced by factors such as pH and temperature, which can affect its reactivity and long-term effects on cellular function. Studies have shown that the modification of enzymes by this compound can lead to progressive changes in enzymatic activity over time . Understanding these temporal effects is essential for optimizing experimental conditions and interpreting results.
Dosage Effects in Animal Models
The effects of this compound vary with different dosages in animal models. At lower doses, the compound may have minimal impact on cellular function, while higher doses can lead to significant changes in enzyme activity and protein function. Toxic or adverse effects may be observed at high doses, highlighting the importance of dosage optimization in experimental studies . These dosage-dependent effects are critical for assessing the compound’s safety and efficacy in biological systems.
Metabolic Pathways
This compound is involved in various metabolic pathways, interacting with enzymes and cofactors that regulate metabolic flux and metabolite levels. The compound’s modification of enzymes such as glutamate dehydrogenase can impact metabolic pathways related to energy production and amino acid metabolism
Transport and Distribution
Within cells and tissues, this compound is transported and distributed through interactions with transporters and binding proteins. These interactions can influence the compound’s localization and accumulation in specific cellular compartments. The transport and distribution of this compound are crucial for understanding its cellular effects and optimizing its use in experimental studies .
Subcellular Localization
The subcellular localization of this compound is determined by targeting signals and post-translational modifications that direct it to specific compartments or organelles. These localization patterns can affect the compound’s activity and function within the cell. Understanding the subcellular localization of this compound is essential for elucidating its mechanism of action and optimizing its use in biochemical research .
properties
IUPAC Name |
4,5,6,7-tetrahydro-2-benzofuran-1,3-dione | |
---|---|---|
Source | PubChem | |
URL | https://pubchem.ncbi.nlm.nih.gov | |
Description | Data deposited in or computed by PubChem | |
InChI |
InChI=1S/C8H8O3/c9-7-5-3-1-2-4-6(5)8(10)11-7/h1-4H2 | |
Source | PubChem | |
URL | https://pubchem.ncbi.nlm.nih.gov | |
Description | Data deposited in or computed by PubChem | |
InChI Key |
HMMBJOWWRLZEMI-UHFFFAOYSA-N | |
Source | PubChem | |
URL | https://pubchem.ncbi.nlm.nih.gov | |
Description | Data deposited in or computed by PubChem | |
Canonical SMILES |
C1CCC2=C(C1)C(=O)OC2=O | |
Source | PubChem | |
URL | https://pubchem.ncbi.nlm.nih.gov | |
Description | Data deposited in or computed by PubChem | |
Molecular Formula |
C8H8O3 | |
Source | PubChem | |
URL | https://pubchem.ncbi.nlm.nih.gov | |
Description | Data deposited in or computed by PubChem | |
DSSTOX Substance ID |
DTXSID9075298 | |
Record name | 1,3-Isobenzofurandione, 4,5,6,7-tetrahydro- | |
Source | EPA DSSTox | |
URL | https://comptox.epa.gov/dashboard/DTXSID9075298 | |
Description | DSSTox provides a high quality public chemistry resource for supporting improved predictive toxicology. | |
Molecular Weight |
152.15 g/mol | |
Source | PubChem | |
URL | https://pubchem.ncbi.nlm.nih.gov | |
Description | Data deposited in or computed by PubChem | |
CAS RN |
2426-02-0 | |
Record name | 3,4,5,6-Tetrahydrophthalic anhydride | |
Source | CAS Common Chemistry | |
URL | https://commonchemistry.cas.org/detail?cas_rn=2426-02-0 | |
Description | CAS Common Chemistry is an open community resource for accessing chemical information. Nearly 500,000 chemical substances from CAS REGISTRY cover areas of community interest, including common and frequently regulated chemicals, and those relevant to high school and undergraduate chemistry classes. This chemical information, curated by our expert scientists, is provided in alignment with our mission as a division of the American Chemical Society. | |
Explanation | The data from CAS Common Chemistry is provided under a CC-BY-NC 4.0 license, unless otherwise stated. | |
Record name | 3,4,5,6-Tetrahydrophthalic acid anhydride | |
Source | ChemIDplus | |
URL | https://pubchem.ncbi.nlm.nih.gov/substance/?source=chemidplus&sourceid=0002426020 | |
Description | ChemIDplus is a free, web search system that provides access to the structure and nomenclature authority files used for the identification of chemical substances cited in National Library of Medicine (NLM) databases, including the TOXNET system. | |
Record name | 2426-02-0 | |
Source | DTP/NCI | |
URL | https://dtp.cancer.gov/dtpstandard/servlet/dwindex?searchtype=NSC&outputformat=html&searchlist=61333 | |
Description | The NCI Development Therapeutics Program (DTP) provides services and resources to the academic and private-sector research communities worldwide to facilitate the discovery and development of new cancer therapeutic agents. | |
Explanation | Unless otherwise indicated, all text within NCI products is free of copyright and may be reused without our permission. Credit the National Cancer Institute as the source. | |
Record name | 2426-02-0 | |
Source | DTP/NCI | |
URL | https://dtp.cancer.gov/dtpstandard/servlet/dwindex?searchtype=NSC&outputformat=html&searchlist=11211 | |
Description | The NCI Development Therapeutics Program (DTP) provides services and resources to the academic and private-sector research communities worldwide to facilitate the discovery and development of new cancer therapeutic agents. | |
Explanation | Unless otherwise indicated, all text within NCI products is free of copyright and may be reused without our permission. Credit the National Cancer Institute as the source. | |
Record name | 1,3-Isobenzofurandione, 4,5,6,7-tetrahydro- | |
Source | EPA Chemicals under the TSCA | |
URL | https://www.epa.gov/chemicals-under-tsca | |
Description | EPA Chemicals under the Toxic Substances Control Act (TSCA) collection contains information on chemicals and their regulations under TSCA, including non-confidential content from the TSCA Chemical Substance Inventory and Chemical Data Reporting. | |
Record name | 1,3-Isobenzofurandione, 4,5,6,7-tetrahydro- | |
Source | EPA DSSTox | |
URL | https://comptox.epa.gov/dashboard/DTXSID9075298 | |
Description | DSSTox provides a high quality public chemistry resource for supporting improved predictive toxicology. | |
Record name | 3,4,5,6-tetrahydrophthalic anhydride | |
Source | European Chemicals Agency (ECHA) | |
URL | https://echa.europa.eu/substance-information/-/substanceinfo/100.017.614 | |
Description | The European Chemicals Agency (ECHA) is an agency of the European Union which is the driving force among regulatory authorities in implementing the EU's groundbreaking chemicals legislation for the benefit of human health and the environment as well as for innovation and competitiveness. | |
Explanation | Use of the information, documents and data from the ECHA website is subject to the terms and conditions of this Legal Notice, and subject to other binding limitations provided for under applicable law, the information, documents and data made available on the ECHA website may be reproduced, distributed and/or used, totally or in part, for non-commercial purposes provided that ECHA is acknowledged as the source: "Source: European Chemicals Agency, http://echa.europa.eu/". Such acknowledgement must be included in each copy of the material. ECHA permits and encourages organisations and individuals to create links to the ECHA website under the following cumulative conditions: Links can only be made to webpages that provide a link to the Legal Notice page. | |
Record name | 3,4,5,6-TETRAHYDROPHTHALIC ANHYDRIDE | |
Source | FDA Global Substance Registration System (GSRS) | |
URL | https://gsrs.ncats.nih.gov/ginas/app/beta/substances/01D3M89A2W | |
Description | The FDA Global Substance Registration System (GSRS) enables the efficient and accurate exchange of information on what substances are in regulated products. Instead of relying on names, which vary across regulatory domains, countries, and regions, the GSRS knowledge base makes it possible for substances to be defined by standardized, scientific descriptions. | |
Explanation | Unless otherwise noted, the contents of the FDA website (www.fda.gov), both text and graphics, are not copyrighted. They are in the public domain and may be republished, reprinted and otherwise used freely by anyone without the need to obtain permission from FDA. Credit to the U.S. Food and Drug Administration as the source is appreciated but not required. | |
Retrosynthesis Analysis
AI-Powered Synthesis Planning: Our tool employs the Template_relevance Pistachio, Template_relevance Bkms_metabolic, Template_relevance Pistachio_ringbreaker, Template_relevance Reaxys, Template_relevance Reaxys_biocatalysis model, leveraging a vast database of chemical reactions to predict feasible synthetic routes.
One-Step Synthesis Focus: Specifically designed for one-step synthesis, it provides concise and direct routes for your target compounds, streamlining the synthesis process.
Accurate Predictions: Utilizing the extensive PISTACHIO, BKMS_METABOLIC, PISTACHIO_RINGBREAKER, REAXYS, REAXYS_BIOCATALYSIS database, our tool offers high-accuracy predictions, reflecting the latest in chemical research and data.
Strategy Settings
Precursor scoring | Relevance Heuristic |
---|---|
Min. plausibility | 0.01 |
Model | Template_relevance |
Template Set | Pistachio/Bkms_metabolic/Pistachio_ringbreaker/Reaxys/Reaxys_biocatalysis |
Top-N result to add to graph | 6 |
Feasible Synthetic Routes
Q & A
A:* Molecular Formula: C8H8O3* Molecular Weight: 152.15 g/mol * Spectroscopic Data: While the provided abstracts don't provide comprehensive spectroscopic data, they mention using changes in UV absorbance at 250 nm to measure the extent of protein modification by 3,4,5,6-tetrahydrophthalic anhydride. [] Further characterization often involves techniques like IR, NMR (both 1H and 13C), and potentially mass spectrometry. [, ]
A: this compound exhibits notable stability under acidic conditions but undergoes hydrolysis at higher pH values. This pH sensitivity has been exploited in the development of pH-responsive nanoparticles for drug delivery applications. For instance, nanoparticles composed of this compound-modified PAMAM dendrimers remain stable at physiological pH (7.4) but readily dissociate in the acidic tumor microenvironment (pH 5.0-6.0), leading to targeted drug release. [, ]
A: While the provided abstracts don't delve into computational studies specifically on this compound, one study mentions using molecular orbital calculations to understand the conformational preferences of the compound in its crystal structure. [] This suggests the possibility of employing computational methods to further explore its structural features, electronic properties, and potential interactions with biological targets. Molecular docking studies have been conducted to predict the binding affinities of this compound and its derivatives with DNA methyltransferase enzymes. []
Disclaimer and Information on In-Vitro Research Products
Please be aware that all articles and product information presented on BenchChem are intended solely for informational purposes. The products available for purchase on BenchChem are specifically designed for in-vitro studies, which are conducted outside of living organisms. In-vitro studies, derived from the Latin term "in glass," involve experiments performed in controlled laboratory settings using cells or tissues. It is important to note that these products are not categorized as medicines or drugs, and they have not received approval from the FDA for the prevention, treatment, or cure of any medical condition, ailment, or disease. We must emphasize that any form of bodily introduction of these products into humans or animals is strictly prohibited by law. It is essential to adhere to these guidelines to ensure compliance with legal and ethical standards in research and experimentation.