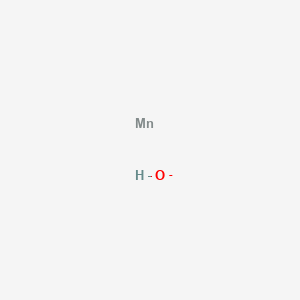
Manganese;hydroxide
- Click on QUICK INQUIRY to receive a quote from our team of experts.
- With the quality product at a COMPETITIVE price, you can focus more on your research.
Overview
Description
Manganese hydroxide, also known as manganese(II) hydroxide, is an inorganic compound with the chemical formula Mn(OH)₂. It typically appears as a white to pale pink solid, although it darkens upon exposure to air due to oxidation. This compound is poorly soluble in water but can dissolve in acids to form manganese(II) salts .
Preparation Methods
Manganese hydroxide can be synthesized in the laboratory by reacting a solution of manganese(II) salt, such as manganese(II) chloride (MnCl₂), with a strong base like sodium hydroxide (NaOH). The reaction produces a precipitate of manganese hydroxide: [ \text{MnCl}_2 (\text{aq}) + 2 \text{NaOH} (\text{aq}) \rightarrow \text{Mn(OH)}_2 (\text{s}) + 2 \text{NaCl} (\text{aq}) ] This synthesis must be handled with care as manganese hydroxide is sensitive to oxidation, which can alter its properties .
Chemical Reactions Analysis
Manganese hydroxide undergoes several types of chemical reactions:
Oxidation: When exposed to air, manganese hydroxide oxidizes to form manganese dioxide (MnO₂)[ 2 \text{Mn(OH)}_2 (\text{s}) + O_2 (\text{g}) \rightarrow 2 \text{MnO}_2 (\text{s}) + 2 \text{H}_2O (\text{l}) ]
Reaction with Acids: Manganese hydroxide reacts with acids to form manganese(II) salts and water[ \text{Mn(OH)}_2 (\text{s}) + 2 \text{HCl} (\text{aq}) \rightarrow \text{MnCl}_2 (\text{aq}) + 2 \text{H}_2O (\text{l}) ]
Reaction with Bases: In the presence of strong bases, manganese hydroxide can form complex ions
Scientific Research Applications
Manganese hydroxide has diverse applications in scientific research and industry:
Battery Production: It serves as a precursor to manganese dioxide (MnO₂), which is crucial in the production of dry cell batteries.
Organic Synthesis: It is used as a reagent in various chemical transformations.
Environmental Science: Manganese hydroxide is employed in removing toxic metals and radionuclides from wastewater through adsorption.
Magnetic Materials:
Zinc-Ion Batteries: Recent research has explored the use of manganese hydroxide nanowire arrays in high-performance zinc-ion batteries.
Mechanism of Action
The mechanism by which manganese hydroxide exerts its effects depends on its application. For instance, in battery production, manganese hydroxide is oxidized to manganese dioxide, which then participates in electrochemical reactions. In environmental applications, manganese hydroxide adsorbs toxic metals and radionuclides, facilitating their removal from wastewater .
Comparison with Similar Compounds
Manganese hydroxide shares similarities with other metal hydroxides, such as magnesium hydroxide (Mg(OH)₂) and calcium hydroxide (Ca(OH)₂). These compounds also adopt the brucite structure, where metal centers are bonded to hydroxide ligands. manganese hydroxide is unique in its specific applications and reactivity. For example, unlike magnesium hydroxide, manganese hydroxide is used as a precursor for manganese dioxide in battery production .
Similar Compounds
- Magnesium hydroxide (Mg(OH)₂)
- Calcium hydroxide (Ca(OH)₂)
- Iron(II) hydroxide (Fe(OH)₂)
- Cobalt(II) hydroxide (Co(OH)₂)
Manganese hydroxide’s distinct properties and applications make it a valuable compound in various scientific and industrial fields.
Properties
Molecular Formula |
HMnO- |
---|---|
Molecular Weight |
71.945 g/mol |
IUPAC Name |
manganese;hydroxide |
InChI |
InChI=1S/Mn.H2O/h;1H2/p-1 |
InChI Key |
BZDIAFGKSAYYFC-UHFFFAOYSA-M |
Canonical SMILES |
[OH-].[Mn] |
Origin of Product |
United States |
Disclaimer and Information on In-Vitro Research Products
Please be aware that all articles and product information presented on BenchChem are intended solely for informational purposes. The products available for purchase on BenchChem are specifically designed for in-vitro studies, which are conducted outside of living organisms. In-vitro studies, derived from the Latin term "in glass," involve experiments performed in controlled laboratory settings using cells or tissues. It is important to note that these products are not categorized as medicines or drugs, and they have not received approval from the FDA for the prevention, treatment, or cure of any medical condition, ailment, or disease. We must emphasize that any form of bodily introduction of these products into humans or animals is strictly prohibited by law. It is essential to adhere to these guidelines to ensure compliance with legal and ethical standards in research and experimentation.