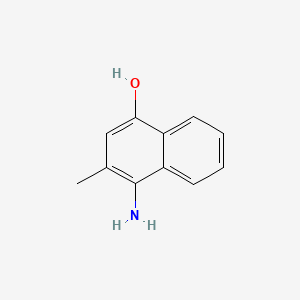
Vitamin K7
- Click on QUICK INQUIRY to receive a quote from our team of experts.
- With the quality product at a COMPETITIVE price, you can focus more on your research.
Overview
Description
Vitamin K7, also known as menaquinone-7, is a member of the vitamin K family, which is essential for various physiological processes in the human body. It is a fat-soluble vitamin that plays a crucial role in bone health and cardiovascular function. This compound is synthesized by certain bacteria in the gut and can also be obtained from dietary sources such as fermented foods like natto, cheese, and sauerkraut .
Preparation Methods
Synthetic Routes and Reaction Conditions: The synthesis of Vitamin K7 involves the attachment of a hexaprenyl chain of “all-trans” configuration to a monoprenyl derivative of menadiol following a “1 + 6” synthetic strategy . This process requires specific reaction conditions to ensure the correct configuration and stability of the compound.
Industrial Production Methods: Industrial production of this compound is primarily achieved through microbial fermentation. Bacillus subtilis, particularly the natto strain, is commonly used for this purpose. The fermentation process involves the use of surfactants to enhance the permeability of the cell membrane, thereby increasing the synthesis of this compound. The addition of 0.7% Tween-80 to the medium has been shown to significantly increase the extracellular and intracellular synthesis of this compound .
Chemical Reactions Analysis
Types of Reactions: Vitamin K7 undergoes various chemical reactions, including oxidation, reduction, and substitution. These reactions are essential for its biological activity and stability.
Common Reagents and Conditions:
Oxidation: this compound can be oxidized using reagents such as potassium permanganate or hydrogen peroxide under controlled conditions.
Reduction: Reduction of this compound can be achieved using reducing agents like sodium borohydride or lithium aluminum hydride.
Substitution: Substitution reactions involving this compound often require catalysts and specific solvents to ensure the desired product formation.
Major Products Formed: The major products formed from these reactions include various derivatives of this compound that retain its biological activity and enhance its stability for pharmaceutical applications .
Scientific Research Applications
Vitamin K7 has a wide range of scientific research applications across various fields:
Chemistry:
- Used as a reagent in organic synthesis to study the effects of different chemical reactions on its structure and activity.
Biology:
- Plays a crucial role in the activation of proteins involved in calcium metabolism, such as osteocalcin and matrix Gla protein .
Medicine:
- Extensively studied for its role in bone health, cardiovascular health, and potential neuroprotective effects. It helps prevent the calcification of arteries and reduces the risk of osteoporosis .
Industry:
Mechanism of Action
Vitamin K7 exerts its effects by activating specific proteins involved in calcium metabolism. One of the key proteins activated by this compound is osteocalcin, which plays a critical role in the mineralization of bone tissue. By activating osteocalcin, this compound ensures that calcium is properly deposited in the bones, promoting bone strength and reducing the risk of osteoporosis .
Additionally, this compound activates matrix Gla protein, which inhibits the deposition of calcium in arterial walls, thereby reducing the risk of arterial stiffness and atherosclerosis .
Comparison with Similar Compounds
Vitamin K1 (Phylloquinone): Primarily found in green leafy vegetables and plays a crucial role in blood clotting.
Menaquinone-4 (MK-4): A subtype of Vitamin K2 with a shorter half-life compared to MK-7.
Uniqueness of Vitamin K7: this compound is unique due to its longer half-life and higher bioavailability compared to other forms of Vitamin K. This allows it to remain in the bloodstream for an extended period, exerting its beneficial effects over a sustained duration .
Properties
CAS No. |
83-69-2 |
---|---|
Molecular Formula |
C11H11NO |
Molecular Weight |
173.21 g/mol |
IUPAC Name |
4-amino-3-methylnaphthalen-1-ol |
InChI |
InChI=1S/C11H11NO/c1-7-6-10(13)8-4-2-3-5-9(8)11(7)12/h2-6,13H,12H2,1H3 |
InChI Key |
YJRCNAAPGQBVFS-UHFFFAOYSA-N |
Canonical SMILES |
CC1=C(C2=CC=CC=C2C(=C1)O)N |
Origin of Product |
United States |
Disclaimer and Information on In-Vitro Research Products
Please be aware that all articles and product information presented on BenchChem are intended solely for informational purposes. The products available for purchase on BenchChem are specifically designed for in-vitro studies, which are conducted outside of living organisms. In-vitro studies, derived from the Latin term "in glass," involve experiments performed in controlled laboratory settings using cells or tissues. It is important to note that these products are not categorized as medicines or drugs, and they have not received approval from the FDA for the prevention, treatment, or cure of any medical condition, ailment, or disease. We must emphasize that any form of bodily introduction of these products into humans or animals is strictly prohibited by law. It is essential to adhere to these guidelines to ensure compliance with legal and ethical standards in research and experimentation.