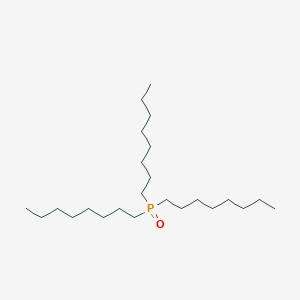
Trioctylphosphine oxide
Overview
Description
Trioctylphosphine oxide (TOPO, (C₈H₁₇)₃PO) is an organophosphorus compound characterized by a central phosphorus atom bonded to three octyl chains and an oxygen atom. It is a white, crystalline solid with a molecular weight of 386.65 g/mol (C₂₄H₅₁OP) and CAS number 78-50-2 . TOPO is highly lipophilic due to its long alkyl chains, making it soluble in nonpolar solvents like toluene and hexane. Its phosphoryl (P=O) group acts as a strong Lewis base, enabling coordination with metal ions .
TOPO is widely used in:
- Nanoparticle synthesis: As a capping agent for CdSe, CdS, and InP quantum dots, stabilizing surfaces and controlling particle size .
- Metal extraction: Efficiently extracting rare earth elements (e.g., Eu³⁺) and uranium from acidic solutions .
- Coordination chemistry: Serving as a ligand in organometallic complexes .
Commercial grades include ReagentPlus® (99%), technical grade (90%), and analytical grade (≥98.5%) .
Preparation Methods
Synthetic Routes for Trioctylphosphine Oxide
TOPO synthesis revolves around phosphorus-based intermediates and alkylation reactions. The primary raw material is sodium hypophosphite (NaH₂PO₂), which undergoes sequential modifications to introduce octyl groups. Three dominant pathways have been industrialized, each differing in reaction sequences and intermediates .
Method 1: Sodium Hypophosphite → Octene Addition → Trichlorosilane Reduction → Bromoalkane Addition → Hydrolysis
This four-step method achieves a 92.6% yield in the final hydrolysis stage .
Octene Addition
Sodium hypophosphite is dissolved in acetic acid and heated to 120°C. Octene is introduced gradually, with the temperature raised to 135°C. The reaction is monitored via ³¹P nuclear magnetic resonance (NMR) until completion. The product, dioctylphosphinic acid (HDOP), is isolated via solvent removal under reduced pressure .
Key Conditions
-
Solvent: Acetic acid
-
Temperature: 120–135°C
-
Monitoring: ³¹P NMR for P–H bond conversion
Trichlorosilane Reduction
HDOP is treated with trichlorosilane (HSiCl₃) in trichloromethane at 80°C for 16 hours. This step reduces phosphinic acid to dioctyl phosphorus chloride (DOPCl), a light green liquid .
Key Conditions
-
Solvent: Trichloromethane
-
Reducing Agent: Trichlorosilane (1:1 molar ratio)
-
Reaction Time: 16 hours
Bromoalkane Addition
DOPCl reacts with bromoalkane (e.g., 1-bromooctane) in toluene at 100°C for 24 hours. This step introduces the third octyl group, forming trioctylphosphine dihalide .
Key Conditions
-
Solvent: Toluene
-
Alkylating Agent: Bromoalkane (excess)
-
Temperature: 100°C
Hydrolysis
The dihalide intermediate undergoes hydrolysis in toluene with distilled water at room temperature. The final product, TOPO, precipitates as a white solid after solvent removal .
Yield Data
Step | Yield (%) |
---|---|
Octene Addition | 90+ |
Trichlorosilane Reduction | 92.9 |
Bromoalkane Addition | 90+ |
Hydrolysis | 92.6 |
Method 2: Sodium Hypophosphite → Octene Addition → Trichlorosilane Reduction → Hydrolysis → Octene Addition
This pathway modifies the alkylation sequence, achieving an 81.4% yield in the final octene addition step .
Hydrolysis of DOPCl
DOPCl is hydrolyzed in trichloromethane with water, yielding dioctyl phosphonic acid. This intermediate is less reactive, requiring stringent temperature control .
Key Conditions
-
Solvent: Trichloromethane
-
Temperature: 25°C
-
Reaction Time: 1 hour
Secondary Octene Addition
Dioctyl phosphonic acid reacts with excess octene in acetic acid at 135°C. The reaction is slower, necessitating 8 hours for completion .
Yield Comparison
-
Hydrolysis Yield: 92.9%
-
Secondary Addition Yield: 81.4%
Method 3: Sodium Hypophosphite → Octene Addition → Trichlorosilane Reduction → Alcoholysis
This streamlined three-step method achieves the highest yield (95.1%) by replacing hydrolysis with alcoholysis .
Alcoholysis of DOPCl
DOPCl reacts with n-octanol in trichloromethane at 50°C for 2 hours. The reaction proceeds via nucleophilic substitution, directly yielding TOPO without dihalide intermediates .
Advantages
-
Fewer steps
-
Higher yield (95.1%)
-
Milder conditions (50°C vs. 100°C)
Reaction Mechanism and Kinetics
Octene Addition: Electrophilic Substitution
The reaction between sodium hypophosphite and octene follows an electrophilic substitution mechanism. The P–H bond in NaH₂PO₂ attacks the electron-deficient β-carbon of octene, forming a P–C bond. Acetic acid stabilizes the transition state, enabling moderate reaction temperatures .
Trichlorosilane Reduction: P=O to P–Cl Conversion
Trichlorosilane reduces phosphinic acid (P=O) to phosphorus chloride (P–Cl). The reaction is exothermic, requiring controlled addition to prevent runaway conditions .
Alcoholysis vs. Hydrolysis: Steric Effects
Alcoholysis benefits from the bulky n-octanol molecule, which minimizes side reactions. In contrast, hydrolysis with water risks forming phosphonic acid byproducts, necessitating precise pH control .
Industrial-Scale Optimization
Solvent Selection
Solvent | Role | Boiling Point (°C) |
---|---|---|
Acetic Acid | Polar aprotic | 118 |
Trichloromethane | Non-polar | 61 |
Toluene | High-boiling | 111 |
Toluene is preferred for high-temperature steps due to its high boiling point and low cost .
Yield Enhancement Strategies
-
Excess Octene : Ensures complete alkylation but requires post-reaction distillation.
-
Inert Atmosphere : Prevents oxidation of phosphorus intermediates.
-
Catalytic Additives : Trace amounts of iodine accelerate alkylation .
Purification and Quality Control
Vacuum Distillation
TOPO is purified via fractional distillation under reduced pressure (400 Pa, 50–65°C). This removes residual solvents and unreacted octene .
³¹P NMR Analysis
³¹P NMR confirms product purity by detecting residual P–H or P–Cl bonds. Commercial TOPO exhibits a single peak at δ 40–45 ppm .
Comparative Analysis of Methods
Parameter | Method 1 | Method 2 | Method 3 |
---|---|---|---|
Total Steps | 4 | 4 | 3 |
Maximum Yield (%) | 92.6 | 81.4 | 95.1 |
Temperature Range | 25–135°C | 25–135°C | 50–135°C |
Byproducts | Dihalide | Phosphonic Acid | None |
Method 3 is superior for industrial applications due to fewer steps and higher yield.
Chemical Reactions Analysis
Types of Reactions: Trioctylphosphine oxide undergoes several types of chemical reactions, including:
Oxidation: Trioctylphosphine reacts with oxygen to form this compound.
Substitution: It can react with various reagents to form different derivatives.
Common Reagents and Conditions:
Oxidizing Agents: Hydrogen peroxide is commonly used for the oxidation of trioctylphosphine.
Solvents: Chloroform and dichloromethane are frequently used as solvents in these reactions.
Major Products:
Scientific Research Applications
Metal Extraction
One of the primary applications of TOPO is in the extraction of metals from aqueous solutions. Its high lipophilicity and polarity enable it to effectively bind to metal ions, making it an excellent extracting agent.
Uranium Extraction
TOPO has been extensively studied for its ability to transport uranium(VI) through liquid membranes. Research indicates that TOPO can facilitate the selective extraction of uranium from dilute solutions, which is crucial for nuclear waste management and recovery processes .
Extraction of Other Metals
TOPO is also employed for extracting other valuable metals such as lanthanides and gallium. A study demonstrated that mixtures of TOPO with carboxylic acids can significantly enhance extraction efficiencies. For instance, a TOPO:malonic acid mixture showed a loading capacity three orders of magnitude greater than conventional methods for gallium extraction from acidic chloride feedstock .
Nanotechnology Applications
TOPO plays a critical role in the synthesis and stabilization of quantum dots, which are semiconductor nanocrystals with unique optical properties.
Quantum Dot Synthesis
TOPO serves as both a solvent and a capping agent during the synthesis of quantum dots, such as CdSe (cadmium selenide). The presence of TOPO stabilizes the growing nanoparticles, preventing agglomeration and ensuring uniform size distribution. This stabilization is essential for achieving desired optical characteristics like photoluminescence .
Enhanced Optical Properties
Research has shown that the use of TOPO in quantum dot synthesis can lead to improved stability and optical features. For example, studies on Sb-alloyed Cs₂NaInCl₆ perovskite nanocrystals highlighted how TOPO-mediated methods enhance both colloidal stability and emission properties .
Development of Functional Liquids
Recent innovations have explored the formulation of hydrophobic functional liquids based on TOPO combined with hydrogen bond donors (HBDs). These mixtures have shown potential as efficient extractants, overcoming solubility limitations typically associated with TOPO in organic solvents.
Deep Eutectic Solvents (DES)
The combination of TOPO with various HBDs has led to the creation of deep eutectic solvents, which exhibit improved extraction capabilities for various solutes from aqueous streams. This approach not only enhances solubility but also increases the efficiency of metal extraction processes .
Case Studies and Research Findings
Mechanism of Action
The mechanism of action of trioctylphosphine oxide is primarily based on its high polarity, which results from the dipolar phosphorus-oxygen bond. This allows the compound to bind effectively to metal ions. The octyl groups confer solubility in low polarity solvents, enhancing its extraction efficiency .
Comparison with Similar Compounds
Comparison with Similar Organophosphorus Compounds
TOPO is part of a broader class of phosphine oxides and organophosphorus extractants. Below is a detailed comparison with structurally related compounds:
Trioctylphosphine (TOP)
- Structure : (C₈H₁₇)₃P (lacks the P=O group).
- Role: Acts as a reducing agent and solvent in nanoparticle synthesis. Unlike TOPO, TOP is a liquid at room temperature .
- Reactivity: TOP oxidizes to TOPO during reactions, influencing nanocrystal growth kinetics .
- Applications : Co-solvent with TOPO in quantum dot synthesis (e.g., CdSe) .
Property | TOPO | TOP |
---|---|---|
Oxidation state of P | +5 (P=O) | +3 (P–C bonds) |
Physical state | Solid | Liquid |
Role in synthesis | Stabilizing agent | Reducing agent/solvent |
Tributylphosphate (TBP)
- Structure : (C₄H₉O)₃PO. Shorter butyl chains vs. TOPO’s octyl chains.
- Extraction efficiency : Less effective than TOPO for Eu³⁺ (log K = 8.2 for TBP vs. 12.5 for TOPO) due to weaker electron-donating alkyl groups .
- Applications : Uranium extraction in nuclear fuel processing; less favored for rare earths due to lower selectivity .
Property | TOPO | TBP |
---|---|---|
Chain length | C₈ (octyl) | C₄ (butyl) |
log K (Eu³⁺ extraction) | 12.5 | 8.2 |
Lipophilicity | Higher | Moderate |
Triphenylphosphine Oxide (TPPO)
- Structure : (C₆H₅)₃PO. Aromatic phenyl groups replace alkyl chains.
- Electronic effects : Phenyl groups are electron-withdrawing, reducing P=O basicity compared to TOPO. Log K for Eu³⁺ extraction = 10.1 .
- Applications : Catalyst in organic synthesis; less common in extraction due to poor solubility in organic phases .
Property | TOPO | TPPO |
---|---|---|
Substituents | Alkyl (electron-donating) | Aryl (electron-withdrawing) |
log K (Eu³⁺ extraction) | 12.5 | 10.1 |
Solubility | High in organic solvents | Low |
Dioctylphosphine Oxide Derivatives
- Example : Sulfur-containing phosphine oxides (e.g., tris(octylthio)phosphine oxide).
- Key difference : Sulfur atoms enhance soft metal (e.g., Hg²⁺, Cd²⁺) extraction but reduce compatibility with aqueous phases vs. TOPO .
Biological Activity
Trioctylphosphine oxide (TOPO) is a phosphine oxide compound widely used in various industrial applications, particularly in the synthesis of nanoparticles and as an extracting agent. Recent research has highlighted its biological activity, particularly regarding its cytotoxic effects, potential use in drug delivery systems, and interactions with biological membranes. This article presents a detailed examination of the biological activity of TOPO, supported by data tables, case studies, and relevant research findings.
TOPO has the chemical formula and is characterized by its hydrophobic nature and ability to form complexes with various metal ions. Its structure includes a phosphine oxide group, which contributes to its reactivity and extraction capabilities in organic solvents.
Biological Activity Overview
The biological activity of TOPO can be categorized into several key areas:
- Cytotoxicity : TOPO has been shown to exhibit cytotoxic effects on various cell lines.
- Antibacterial Properties : It has moderate antibacterial activity against certain bacterial strains.
- Nanoparticle Synthesis : TOPO plays a significant role in the synthesis of nanoparticles, influencing their size and morphology.
Cytotoxicity Studies
Recent studies have explored the cytotoxic effects of TOPO on cancer cell lines. For example, a study assessed the impact of TOPO on human cervical carcinoma (HeLa) cells and endometrial adenocarcinoma (Ishikawa) cells using the MTT assay. The findings indicated that TOPO exhibited significant cytotoxicity with half-maximal inhibitory concentration (IC50) values ranging from 10 to 50 µM, depending on the exposure time and cell line used.
Table 1: IC50 Values for TOPO Against Various Cell Lines
Cell Line | IC50 (µM) | Exposure Time (h) |
---|---|---|
HeLa | 25 | 24 |
Ishikawa | 30 | 24 |
Non-tumorigenic L929 Cells | 100 | 24 |
The mechanism underlying TOPO's cytotoxicity appears to involve the induction of reactive oxygen species (ROS) and disruption of cellular membranes. Studies have shown that treatment with TOPO leads to increased ROS levels, which can cause oxidative stress and subsequent apoptosis in cancer cells. Additionally, flow cytometry analysis indicated that TOPO treatment resulted in cell cycle arrest predominantly at the S phase, suggesting interference with DNA replication processes.
Antibacterial Activity
TOPO's antibacterial properties have also been investigated. A study reported that TOPO demonstrated moderate efficacy against Staphylococcus aureus and Escherichia coli. The minimal inhibitory concentration (MIC) values were determined to be around 200 µg/mL for both bacterial strains.
Table 2: Antibacterial Activity of TOPO
Bacterial Strain | MIC (µg/mL) |
---|---|
Staphylococcus aureus | 200 |
Escherichia coli | 200 |
Applications in Nanotechnology
TOPO is extensively used in nanoparticle synthesis due to its ability to stabilize metal precursors during reduction reactions. For instance, in the synthesis of cadmium selenide (CdSe) quantum dots, TOPO serves as a coordinating ligand that controls particle size and distribution. The resulting nanoparticles exhibit unique optical properties suitable for applications in photonics and bioimaging.
Case Study: Synthesis of CdSe Quantum Dots
In a controlled study, varying concentrations of TOPO were employed to synthesize CdSe quantum dots at different temperatures. The results indicated that increasing TOPO concentrations led to larger particle sizes and enhanced photoluminescence properties.
Q & A
Basic Research Questions
Q. What are the key physicochemical properties of TOPO, and how do they influence its role as a solvent or ligand in coordination chemistry?
TOPO is a solid organophosphorus compound (melting point: 52–55°C) with the molecular formula C24H51OP. Its high hydrophobicity and strong electron-donating ability stem from the three octyl chains and phosphoryl oxygen, enabling it to act as a ligand for transition metals and lanthanides. The octyl groups provide steric bulk, influencing selectivity in metal coordination . Purity grades (90–99%) should be verified via HPLC or GC to ensure reproducibility in solvent-dependent reactions .
Q. How can researchers purify TOPO to minimize impurities that affect experimental outcomes?
Technical-grade TOPO (90% purity) often contains residual phosphines or oxidation byproducts. Recrystallization from hot ethanol or hexane is recommended. Purity validation should include <sup>31</sup>P NMR to detect phosphine impurities (δ ~20 ppm for TOPO vs. δ ~-5 ppm for trioctylphosphine) and elemental analysis for phosphorus content .
Q. What analytical techniques are suitable for characterizing TOPO and its metal complexes?
- <sup>31</sup>P NMR : Detects coordination shifts (e.g., δ ~30 ppm for free TOPO vs. δ ~40 ppm when bound to lanthanides) .
- FT-IR : The P=O stretch (~1150–1200 cm<sup>-1</sup>) shifts upon hydrogen bonding or metal coordination .
- X-ray absorption spectroscopy (XAS) : Resolves bonding behavior in lanthanide-TOPO complexes by probing 4f orbital contributions .
Advanced Research Questions
Q. How does TOPO’s structure influence its efficacy in liquid-liquid extraction systems, particularly for rare-earth elements?
TOPO’s extraction efficiency correlates with its ability to form stable complexes via phosphoryl oxygen-metal interactions. In nitric acid media, TOPO extracts UO2<sup>2+</sup> and lanthanides through solvation mechanisms. Competitive extraction studies using varying nitrate concentrations and pH can optimize selectivity. Density functional theory (DFT) calculations predict binding energies and ligand geometry effects .
Q. What experimental strategies resolve contradictions in reported coordination stoichiometries of TOPO-metal complexes?
Discrepancies in stoichiometry (e.g., 1:3 vs. 1:4 metal:TOPO ratios) arise from solvent polarity and counterion effects. Use Job’s method (continuous variation) in non-polar solvents (e.g., toluene) to determine predominant species. Complement with ESI-MS to detect ionized complexes and X-ray crystallography for unambiguous structural assignment .
Q. How can researchers mitigate batch-to-batch variability in TOPO-driven nanoparticle synthesis?
TOPO’s role as a surfactant in nanoparticle synthesis is sensitive to trace water or phosphine impurities. Pre-treatment with molecular sieves and degassing under vacuum reduces variability. Monitor reaction kinetics via in-situ UV-Vis spectroscopy to correlate TOPO purity with nucleation rates .
Q. What computational approaches elucidate TOPO’s role in asymmetric catalysis or chiral recognition?
Molecular dynamics (MD) simulations can model TOPO’s interaction with chiral substrates, focusing on steric effects from octyl chains. Pair with quantum mechanical calculations (e.g., DFT) to map electrostatic potential surfaces and predict enantioselectivity trends. Experimental validation via <sup>1</sup>H NMR titration with chiral shift reagents is advised .
Q. Methodological Considerations Table
Properties
IUPAC Name |
1-dioctylphosphoryloctane | |
---|---|---|
Source | PubChem | |
URL | https://pubchem.ncbi.nlm.nih.gov | |
Description | Data deposited in or computed by PubChem | |
InChI |
InChI=1S/C24H51OP/c1-4-7-10-13-16-19-22-26(25,23-20-17-14-11-8-5-2)24-21-18-15-12-9-6-3/h4-24H2,1-3H3 | |
Source | PubChem | |
URL | https://pubchem.ncbi.nlm.nih.gov | |
Description | Data deposited in or computed by PubChem | |
InChI Key |
ZMBHCYHQLYEYDV-UHFFFAOYSA-N | |
Source | PubChem | |
URL | https://pubchem.ncbi.nlm.nih.gov | |
Description | Data deposited in or computed by PubChem | |
Canonical SMILES |
CCCCCCCCP(=O)(CCCCCCCC)CCCCCCCC | |
Source | PubChem | |
URL | https://pubchem.ncbi.nlm.nih.gov | |
Description | Data deposited in or computed by PubChem | |
Molecular Formula |
C24H51OP | |
Source | PubChem | |
URL | https://pubchem.ncbi.nlm.nih.gov | |
Description | Data deposited in or computed by PubChem | |
DSSTOX Substance ID |
DTXSID1052537 | |
Record name | Trioctylphosphine oxide | |
Source | EPA DSSTox | |
URL | https://comptox.epa.gov/dashboard/DTXSID1052537 | |
Description | DSSTox provides a high quality public chemistry resource for supporting improved predictive toxicology. | |
Molecular Weight |
386.6 g/mol | |
Source | PubChem | |
URL | https://pubchem.ncbi.nlm.nih.gov | |
Description | Data deposited in or computed by PubChem | |
Physical Description |
White solid; [Sigma-Aldrich MSDS] | |
Record name | Trioctylphosphine oxide | |
Source | Haz-Map, Information on Hazardous Chemicals and Occupational Diseases | |
URL | https://haz-map.com/Agents/18395 | |
Description | Haz-Map® is an occupational health database designed for health and safety professionals and for consumers seeking information about the adverse effects of workplace exposures to chemical and biological agents. | |
Explanation | Copyright (c) 2022 Haz-Map(R). All rights reserved. Unless otherwise indicated, all materials from Haz-Map are copyrighted by Haz-Map(R). No part of these materials, either text or image may be used for any purpose other than for personal use. Therefore, reproduction, modification, storage in a retrieval system or retransmission, in any form or by any means, electronic, mechanical or otherwise, for reasons other than personal use, is strictly prohibited without prior written permission. | |
CAS No. |
78-50-2 | |
Record name | Trioctylphosphine oxide | |
Source | CAS Common Chemistry | |
URL | https://commonchemistry.cas.org/detail?cas_rn=78-50-2 | |
Description | CAS Common Chemistry is an open community resource for accessing chemical information. Nearly 500,000 chemical substances from CAS REGISTRY cover areas of community interest, including common and frequently regulated chemicals, and those relevant to high school and undergraduate chemistry classes. This chemical information, curated by our expert scientists, is provided in alignment with our mission as a division of the American Chemical Society. | |
Explanation | The data from CAS Common Chemistry is provided under a CC-BY-NC 4.0 license, unless otherwise stated. | |
Record name | Trioctyl phosphine oxide | |
Source | ChemIDplus | |
URL | https://pubchem.ncbi.nlm.nih.gov/substance/?source=chemidplus&sourceid=0000078502 | |
Description | ChemIDplus is a free, web search system that provides access to the structure and nomenclature authority files used for the identification of chemical substances cited in National Library of Medicine (NLM) databases, including the TOXNET system. | |
Record name | Trioctylphosphine oxide | |
Source | DTP/NCI | |
URL | https://dtp.cancer.gov/dtpstandard/servlet/dwindex?searchtype=NSC&outputformat=html&searchlist=41937 | |
Description | The NCI Development Therapeutics Program (DTP) provides services and resources to the academic and private-sector research communities worldwide to facilitate the discovery and development of new cancer therapeutic agents. | |
Explanation | Unless otherwise indicated, all text within NCI products is free of copyright and may be reused without our permission. Credit the National Cancer Institute as the source. | |
Record name | Phosphine oxide, trioctyl- | |
Source | EPA Chemicals under the TSCA | |
URL | https://www.epa.gov/chemicals-under-tsca | |
Description | EPA Chemicals under the Toxic Substances Control Act (TSCA) collection contains information on chemicals and their regulations under TSCA, including non-confidential content from the TSCA Chemical Substance Inventory and Chemical Data Reporting. | |
Record name | Trioctylphosphine oxide | |
Source | EPA DSSTox | |
URL | https://comptox.epa.gov/dashboard/DTXSID1052537 | |
Description | DSSTox provides a high quality public chemistry resource for supporting improved predictive toxicology. | |
Record name | Trioctylphosphine oxide | |
Source | European Chemicals Agency (ECHA) | |
URL | https://echa.europa.eu/substance-information/-/substanceinfo/100.001.020 | |
Description | The European Chemicals Agency (ECHA) is an agency of the European Union which is the driving force among regulatory authorities in implementing the EU's groundbreaking chemicals legislation for the benefit of human health and the environment as well as for innovation and competitiveness. | |
Explanation | Use of the information, documents and data from the ECHA website is subject to the terms and conditions of this Legal Notice, and subject to other binding limitations provided for under applicable law, the information, documents and data made available on the ECHA website may be reproduced, distributed and/or used, totally or in part, for non-commercial purposes provided that ECHA is acknowledged as the source: "Source: European Chemicals Agency, http://echa.europa.eu/". Such acknowledgement must be included in each copy of the material. ECHA permits and encourages organisations and individuals to create links to the ECHA website under the following cumulative conditions: Links can only be made to webpages that provide a link to the Legal Notice page. | |
Record name | TRIOCTYLPHOSPHINE OXIDE | |
Source | FDA Global Substance Registration System (GSRS) | |
URL | https://gsrs.ncats.nih.gov/ginas/app/beta/substances/EXU8U2AM5A | |
Description | The FDA Global Substance Registration System (GSRS) enables the efficient and accurate exchange of information on what substances are in regulated products. Instead of relying on names, which vary across regulatory domains, countries, and regions, the GSRS knowledge base makes it possible for substances to be defined by standardized, scientific descriptions. | |
Explanation | Unless otherwise noted, the contents of the FDA website (www.fda.gov), both text and graphics, are not copyrighted. They are in the public domain and may be republished, reprinted and otherwise used freely by anyone without the need to obtain permission from FDA. Credit to the U.S. Food and Drug Administration as the source is appreciated but not required. | |
Synthesis routes and methods
Procedure details
Retrosynthesis Analysis
AI-Powered Synthesis Planning: Our tool employs the Template_relevance Pistachio, Template_relevance Bkms_metabolic, Template_relevance Pistachio_ringbreaker, Template_relevance Reaxys, Template_relevance Reaxys_biocatalysis model, leveraging a vast database of chemical reactions to predict feasible synthetic routes.
One-Step Synthesis Focus: Specifically designed for one-step synthesis, it provides concise and direct routes for your target compounds, streamlining the synthesis process.
Accurate Predictions: Utilizing the extensive PISTACHIO, BKMS_METABOLIC, PISTACHIO_RINGBREAKER, REAXYS, REAXYS_BIOCATALYSIS database, our tool offers high-accuracy predictions, reflecting the latest in chemical research and data.
Strategy Settings
Precursor scoring | Relevance Heuristic |
---|---|
Min. plausibility | 0.01 |
Model | Template_relevance |
Template Set | Pistachio/Bkms_metabolic/Pistachio_ringbreaker/Reaxys/Reaxys_biocatalysis |
Top-N result to add to graph | 6 |
Feasible Synthetic Routes
Disclaimer and Information on In-Vitro Research Products
Please be aware that all articles and product information presented on BenchChem are intended solely for informational purposes. The products available for purchase on BenchChem are specifically designed for in-vitro studies, which are conducted outside of living organisms. In-vitro studies, derived from the Latin term "in glass," involve experiments performed in controlled laboratory settings using cells or tissues. It is important to note that these products are not categorized as medicines or drugs, and they have not received approval from the FDA for the prevention, treatment, or cure of any medical condition, ailment, or disease. We must emphasize that any form of bodily introduction of these products into humans or animals is strictly prohibited by law. It is essential to adhere to these guidelines to ensure compliance with legal and ethical standards in research and experimentation.