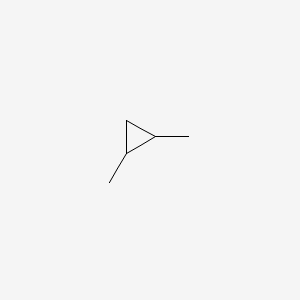
1,2-Dimethylcyclopropane
- Click on QUICK INQUIRY to receive a quote from our team of experts.
- With the quality product at a COMPETITIVE price, you can focus more on your research.
Overview
Description
1,2-Dimethylcyclopropane is a cycloalkane consisting of a cyclopropane ring substituted with two methyl groups attached to adjacent carbon atoms. It has three stereoisomers: one cis-isomer and a pair of trans-enantiomers, which differ depending on the orientation of the two methyl groups . The compound is known for its ring tension, which results in a relatively unstable structure .
Preparation Methods
Synthetic Routes and Reaction Conditions
1,2-Dimethylcyclopropane can be synthesized through the reaction of carbenes with alkenes. One common method involves the use of dichlorocarbene, which is generated in situ from chloroform and potassium hydroxide . The reaction proceeds as follows: [ \text{CHCl}_3 + \text{KOH} \rightarrow \text{CCl}_2 + \text{KCl} + \text{H}_2\text{O} ] [ \text{CCl}_2 + \text{Alkene} \rightarrow \text{Cyclopropane derivative} ]
Industrial Production Methods
Industrial production of this compound typically involves the use of specialized reactors to control the reaction conditions, such as temperature and pressure, to optimize yield and purity. The process may also involve the use of catalysts to enhance the reaction rate and selectivity.
Chemical Reactions Analysis
Types of Reactions
1,2-Dimethylcyclopropane undergoes various types of chemical reactions, including:
Oxidation: The compound can be oxidized to form corresponding alcohols, ketones, or carboxylic acids.
Reduction: Reduction reactions can convert the compound into simpler hydrocarbons.
Substitution: Halogenation reactions can introduce halogen atoms into the cyclopropane ring.
Common Reagents and Conditions
Oxidation: Common oxidizing agents include potassium permanganate (KMnO₄) and chromium trioxide (CrO₃).
Reduction: Hydrogen gas (H₂) in the presence of a palladium catalyst (Pd/C) is often used.
Substitution: Halogenation can be carried out using halogens like chlorine (Cl₂) or bromine (Br₂) under UV light.
Major Products Formed
Oxidation: Alcohols, ketones, and carboxylic acids.
Reduction: Alkanes.
Substitution: Halogenated cyclopropanes.
Scientific Research Applications
1,2-Dimethylcyclopropane has various applications in scientific research:
Chemistry: Used as a model compound to study the reactivity and stability of cyclopropane derivatives.
Biology: Investigated for its potential effects on biological systems, including enzyme interactions.
Medicine: Explored for its potential use in drug development and as a building block for pharmaceutical compounds.
Industry: Utilized in the synthesis of specialty chemicals and materials.
Mechanism of Action
The mechanism of action of 1,2-Dimethylcyclopropane involves its interaction with molecular targets through its strained ring structure. The ring tension makes the compound highly reactive, allowing it to participate in various chemical reactions. The pathways involved often include the formation of reactive intermediates, such as carbenes, which can further react with other molecules .
Comparison with Similar Compounds
1,2-Dimethylcyclopropane is one of several structural isomers with the formula C₅H₁₀. Similar compounds include:
- Cyclopentane
- Methylcyclobutane
- 1,1-Dimethylcyclopropane
- Ethylcyclopropane
- 1-Pentene
- 2-Pentene
- 2-Methyl-1-butene
- 3-Methyl-1-butene
- 2-Methyl-2-butene
Compared to these compounds, this compound is unique due to its specific ring structure and the presence of two adjacent methyl groups, which contribute to its distinct chemical properties and reactivity.
Properties
CAS No. |
2511-95-7 |
---|---|
Molecular Formula |
C5H10 |
Molecular Weight |
70.13 g/mol |
IUPAC Name |
1,2-dimethylcyclopropane |
InChI |
InChI=1S/C5H10/c1-4-3-5(4)2/h4-5H,3H2,1-2H3 |
InChI Key |
VKJLDXGFBJBTRQ-UHFFFAOYSA-N |
Canonical SMILES |
CC1CC1C |
Origin of Product |
United States |
Disclaimer and Information on In-Vitro Research Products
Please be aware that all articles and product information presented on BenchChem are intended solely for informational purposes. The products available for purchase on BenchChem are specifically designed for in-vitro studies, which are conducted outside of living organisms. In-vitro studies, derived from the Latin term "in glass," involve experiments performed in controlled laboratory settings using cells or tissues. It is important to note that these products are not categorized as medicines or drugs, and they have not received approval from the FDA for the prevention, treatment, or cure of any medical condition, ailment, or disease. We must emphasize that any form of bodily introduction of these products into humans or animals is strictly prohibited by law. It is essential to adhere to these guidelines to ensure compliance with legal and ethical standards in research and experimentation.