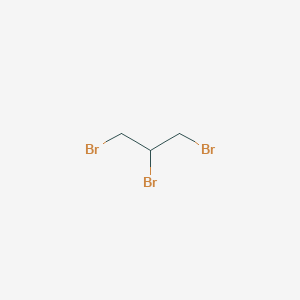
1,2,3-Tribromopropane
Overview
Description
1,2,3-Tribromopropane (CAS 96-11-7) is a brominated alkane with the molecular formula C₃H₅Br₃ and a molecular weight of 280.78 g/mol . It is a volatile organic compound (VOC) historically used as a nematocide in agriculture and as a chemical intermediate in organic synthesis . Its physical properties include a melting point of 16–24°C, a density of 2.38 g/cm³, and a liquid state at room temperature . Due to its toxicity and environmental persistence, its industrial use has declined, though it remains a subject of research in biodegradation and environmental remediation .
Preparation Methods
Synthetic Routes and Reaction Conditions
Bromination of Allyl Bromide
The addition of bromine (Br₂) to allyl bromide (C₃H₅Br) is a well-documented method for synthesizing 1,2,3-tribromopropane. This reaction typically occurs in a non-polar solvent such as carbon tetrachloride (CCl₄) under controlled temperature conditions. The exothermic nature of bromine addition necessitates gradual mixing and cooling to prevent runaway reactions.
Key Reaction Parameters:
-
Molar Ratio: A 3:1 stoichiometric ratio of Br₂ to allyl bromide ensures complete tribromination.
-
Temperature: Maintained between 0–5°C to moderate reaction kinetics.
-
Solvent: CCl₄ or dichloromethane (DCM) enhances solubility and heat dissipation.
This method yields this compound with a purity exceeding 90%, though post-reaction purification via fractional distillation is often required to remove excess bromine and byproducts such as 1,2-dibromopropane .
Halogen Exchange Reactions
Phosphorus pentabromide (PBr₅) can facilitate halogen exchange in epibromohydrin or symmetrical dibromohydrin. For example, treating epibromohydrin (C₃H₅BrO) with PBr₅ at elevated temperatures (80–100°C) replaces the hydroxyl group with a bromine atom, yielding this compound.
Mechanistic Insights:
-
PBr₅ acts as a Lewis acid, polarizing the C–O bond in epibromohydrin.
-
The reaction proceeds via an SN2 mechanism, with bromide ions displacing the hydroxyl group.
This route is less common industrially due to the high cost of PBr₅ and challenges in handling hygroscopic reagents .
Hydrobromination of 1,3-Dibromopropene
The addition of hydrogen bromide (HBr) to 1,3-dibromopropene (C₃H₄Br₂) under acidic conditions provides a regioselective pathway to this compound. The reaction follows Markovnikov’s rule, with HBr adding to the less substituted double-bond carbon.
Optimization Considerations:
-
Catalyst: FeBr₃ or AlBr₃ enhances reaction rate and selectivity.
-
Solvent: Hydrobromic acid (48% w/w) serves as both solvent and reactant.
This method is advantageous for its high atom economy but requires stringent control over reaction conditions to avoid polymerization of the alkene .
Industrial Production Methods
Continuous-Flow Bromination
Industrial-scale synthesis often employs continuous-flow reactors to improve safety and efficiency. Allyl bromide and bromine are fed into a reactor equipped with static mixers and cooling jackets, ensuring precise temperature control and rapid mixing.
Advantages:
-
Minimizes thermal degradation of products.
-
Scalable to multi-ton production capacities.
Challenges:
-
Corrosion-resistant materials (e.g., Hastelloy) are required due to bromine’s reactivity.
-
Waste management of HBr byproducts necessitates scrubbing systems .
Purification and Quality Control
Crude this compound is purified via vacuum distillation to achieve pharmaceutical-grade purity (>99%). Gas chromatography (GC) and nuclear magnetic resonance (NMR) spectroscopy are standard analytical techniques for verifying composition and detecting impurities such as residual allyl bromide or dibromopropanes .
Comparative Analysis of Synthetic Routes
Method | Yield (%) | Purity (%) | Cost (Relative) | Scalability |
---|---|---|---|---|
Bromination of Allyl Br | 85–90 | 90–95 | Low | High |
Halogen Exchange | 70–75 | 80–85 | High | Moderate |
Hydrobromination | 80–85 | 88–92 | Moderate | High |
Chemical Reactions Analysis
Types of Reactions: 1,2,3-Tribromopropane undergoes several types of chemical reactions, including:
Substitution Reactions: It can undergo nucleophilic substitution reactions where the bromine atoms are replaced by other nucleophiles.
Elimination Reactions: It can undergo elimination reactions to form alkenes or alkynes.
Common Reagents and Conditions:
Nucleophiles: Common nucleophiles used in substitution reactions include hydroxide ions, alkoxide ions, and amines.
Bases: Strong bases like potassium tert-butoxide are used in elimination reactions.
Major Products:
Substitution Products: Depending on the nucleophile, the major products can include alcohols, ethers, or amines.
Elimination Products: The major products of elimination reactions are alkenes or alkynes.
Scientific Research Applications
Organic Synthesis
1,2,3-Tribromopropane is widely utilized as a reagent in organic synthesis. Its ability to act as an electrophile makes it valuable for the preparation of complex organic molecules. It serves as an intermediate in synthesizing pharmaceuticals and agrochemicals, facilitating advancements in these fields .
Flame Retardants
One of the most significant applications of this compound is in the production of flame retardants. It enhances the fire safety of materials used in textiles and plastics by reducing their flammability. This application is crucial in consumer products such as furniture and electronics, where fire safety is paramount .
Solvent Applications
The compound also functions as a solvent in various chemical reactions and processes. Its use as a solvent is particularly beneficial in laboratory settings for synthesizing other compounds and conducting chemical analyses .
Analytical Chemistry
In analytical chemistry, this compound is employed as a standard for calibrating instruments and validating methods. This ensures accuracy in measurements and enhances the reliability of analytical results .
Biological Studies
Research has explored the biological effects of this compound, particularly its mutagenicity and potential toxicity. Studies have indicated that exposure can lead to adverse effects on genetic material and various organ systems . Its structural similarity to other brominated compounds raises concerns regarding reproductive toxicity and carcinogenic potential .
Environmental Impact Research
The environmental behavior of this compound has become an area of significant interest due to its potential persistence and bioaccumulation in ecosystems. Research focuses on understanding how brominated compounds degrade in the environment and their long-term ecological effects . Investigations into its toxicity to aquatic organisms are also critical for assessing its environmental risks.
Case Studies
Several case studies have highlighted the applications and implications of this compound:
- Flame Retardant Efficacy : A study demonstrated that incorporating TBP into polymer matrices significantly improved their fire resistance compared to untreated materials. This research underlines its effectiveness as a flame retardant in commercial products.
- Toxicological Assessments : Laboratory studies on rodents exposed to varying concentrations of TBP revealed acute toxicity levels that raised concerns about its safety profile. These findings are crucial for regulatory assessments regarding its use in consumer products.
- Environmental Degradation Studies : Research examining the degradation pathways of TBP in aquatic environments indicated that it could form reactive metabolites that may pose risks to marine life. This study emphasizes the need for careful evaluation before widespread application.
Mechanism of Action
The mechanism of action of 1,2,3-tribromopropane involves its ability to undergo nucleophilic substitution and elimination reactions. The bromine atoms in the compound are highly reactive and can be replaced by other nucleophiles or eliminated to form double or triple bonds. This reactivity makes it a valuable reagent in organic synthesis .
Comparison with Similar Compounds
Comparison with Structurally Similar Compounds
1,2-Dibromo-3-Chloropropane (DBCP)
- Chemical Structure : C₃H₅Br₂Cl
- Key Differences: Toxicity: DBCP exhibits higher genotoxicity and carcinogenicity compared to 1,2,3-tribromopropane. It was banned in the U.S. in 1979 due to its role in causing infertility and cancer . Biodegradability: Both compounds are degraded by the enzyme haloalkane dehalogenase (DhaA), but this compound has a lower Km (0.14 mM) and higher kcat (4.6 s⁻¹) than DBCP (Km = 0.25 mM, kcat = 3.5 s⁻¹), indicating faster enzymatic processing . Environmental Impact: DBCP is a more persistent groundwater contaminant, with slower degradation rates due to its mixed halogenation .
1,2,3-Trichloropropane (TCP)
- Chemical Structure : C₃H₅Cl₃
- Key Differences :
- Synthesis : TCP is a by-product of epichlorohydrin production, unlike this compound, which is synthesized via bromination of allyl bromide or glycerol .
- Enzyme Affinity : TCP is a poor substrate for DhaA, with a Km > 10 mM and kcat = 0.03 s⁻¹ , making it resistant to microbial degradation compared to its brominated counterpart .
- Physical Properties : TCP has a lower density (1.39 g/cm³) and higher volatility , contributing to its widespread detection in air and water .
1,1,3-Tribromopropane
- Chemical Structure : C₃H₅Br₃ (isomeric form)
- Key Differences: Toxicity Profile: 1,1,3-Tribromopropane shows lower mutagenic activity in Salmonella assays compared to this compound, likely due to steric effects altering DNA interaction . Applications: Limited industrial use, primarily studied for comparative toxicology rather than commercial applications .
1,1,2-Tribromopropane
- Chemical Structure : C₃H₅Br₃ (isomeric form)
- Key Differences: Synthesis: Produced via alternative bromination pathways, such as bromine addition to propene derivatives . Environmental Behavior: Limited data, but its branched structure may reduce enzymatic recognition compared to linear this compound .
Data Tables
Table 1. Physical and Chemical Properties
Table 2. Enzymatic Degradation Parameters (DhaA)
Compound | Km (mM) | kcat (s⁻¹) | Relative Efficiency | |
---|---|---|---|---|
This compound | 0.14 | 4.6 | 100% | |
1,2-Dibromo-3-chloropropane | 0.25 | 3.5 | 58% | |
1,2,3-Trichloropropane | >10 | 0.03 | <1% |
Environmental and Toxicological Comparison
- Persistence : Brominated propanes generally degrade faster than chlorinated analogs due to higher enzyme affinity, but their volatility increases atmospheric dispersal .
- Genotoxicity: this compound induces dominant lethal mutations in rats, while DBCP causes testicular atrophy and carcinogenicity .
- Regulatory Status : DBCP is banned globally, whereas this compound faces restrictions in the EU under REACH regulations .
Biological Activity
1,2,3-Tribromopropane (TBP) is a brominated organic compound that has been studied for its biological activity, particularly in the context of environmental toxicity and potential applications in bioremediation. This article provides a detailed overview of the biological effects of TBP, supported by data tables, case studies, and research findings from diverse sources.
This compound is a colorless liquid with a molecular formula of C₃H₃Br₃. It is known for its high reactivity due to the presence of three bromine atoms, which can significantly influence its interaction with biological systems.
Toxicity and Environmental Impact
- Toxicological Studies : Research has shown that TBP exhibits significant toxicity to various organisms. For instance, studies on aquatic life have demonstrated that TBP can adversely affect fish and invertebrate populations. The compound's toxicity is primarily attributed to its ability to disrupt cellular functions and induce oxidative stress.
- Genotoxicity : A study comparing the genotoxic effects of TBP with other brominated compounds indicated that TBP has mutagenic properties. In vitro assays showed that TBP could cause DNA damage in bacterial strains such as Salmonella typhimurium, suggesting its potential carcinogenicity .
- Metabolic Pathways : The metabolism of TBP in laboratory animals reveals that it undergoes biotransformation resulting in various metabolites, some of which may retain toxic properties. Studies indicate that the compound can covalently bind to macromolecules in cells, leading to long-term biological effects .
Case Studies
-
Case Study 1: Aquatic Toxicity
A study conducted on the effects of TBP on freshwater organisms revealed a concentration-dependent mortality rate among fish species exposed to varying levels of TBP over a 48-hour period. The lethal concentration (LC50) was determined to be approximately 5 mg/L for certain sensitive species. -
Case Study 2: Genotoxic Effects
In an experimental setup involving Salmonella strains, TBP was found to induce mutations at concentrations as low as 10 µg/mL. This finding underscores the importance of monitoring brominated compounds in environmental assessments due to their potential risks to human health and ecosystems .
Enzymatic Activity and Bioremediation Potential
Recent investigations into haloalkane dehalogenases have highlighted their role in the biodegradation of halogenated compounds like TBP. Enzymes such as DhaA from Rhodococcus species have shown promise in catalyzing the dehalogenation of TBP, thus facilitating its breakdown into less harmful substances.
Enzyme | Substrate | kcat (s⁻¹) | KM (µM) |
---|---|---|---|
DhaA | This compound | 0.5 | 100 |
DhlA | 1,2-Dibromopropane | 1.5 | 50 |
This table summarizes kinetic parameters for various dehalogenases acting on brominated substrates, indicating that while DhaA shows lower activity on TBP compared to other substrates, it still presents a viable option for bioremediation strategies .
Q & A
Basic Research Questions
Q. What are the established synthesis methods for 1,2,3-Tribromopropane, and how do reaction conditions influence yield and purity?
The primary synthesis involves the bromination of propane or propene derivatives under controlled conditions. For example, radical bromination of propene using bromine gas in the presence of UV light typically produces a mixture of brominated alkanes, requiring fractional distillation to isolate this compound. Reaction temperature (optimized at 40–60°C) and stoichiometric ratios of bromine to precursor are critical for minimizing byproducts like 1,2-dibromopropane . Purity (>97%) is confirmed via gas chromatography (GC), as noted in reagent catalogs .
Q. What analytical techniques are recommended for characterizing this compound’s physicochemical properties?
Key techniques include:
- GC-MS : For quantifying purity and identifying volatile impurities .
- NMR spectroscopy : To confirm molecular structure (e.g., distinct signals for three bromine atoms on adjacent carbons in H and C NMR) .
- DSC/TGA : To determine thermal stability (decomposition onset at ~205°C) and melting/boiling points (128–205°C) .
Q. What are the acute toxicological effects of this compound, and how should they inform laboratory safety protocols?
Studies classify it as a skin/eye irritant and potential carcinogen. Acute exposure in rodents shows LD values of 200–300 mg/kg (oral) and 450–600 mg/kg (dermal). Researchers must use fume hoods, nitrile gloves, and emergency eyewash stations. Storage should avoid light and moisture to prevent decomposition into hydrogen bromide .
Advanced Research Questions
Q. How can experimental designs be optimized to study this compound’s environmental degradation pathways?
Use Agrobacterium radiobacter strain AD1(pTB3) to model biodegradation. Key steps include:
- Dehalogenation : Monitor enzymatic conversion to 2,3-dibromo-1-propanol via haloalkane dehalogenase.
- Epoxidation : Track epoxide hydrolase activity producing 3-bromo-1,2-propanediol.
- Metabolite analysis : Employ LC-MS to identify intermediates like glycerol . Control variables: pH (6.5–7.5), temperature (25–30°C), and oxygen levels for aerobic conditions .
Q. How should researchers address contradictions in reported toxicity data for this compound across studies?
Discrepancies often arise from differences in:
- Exposure duration : Acute vs. chronic studies (e.g., conflicting carcinogenicity reports).
- Model organisms : Variations in metabolic pathways between rodents and aquatic species.
- Purity of samples : Impurities like residual bromine may skew results. Mitigation: Replicate studies using standardized OECD guidelines and cross-validate with in vitro assays (e.g., Ames test for mutagenicity) .
Q. What methodologies are effective for assessing this compound’s ecotoxicity in aquatic ecosystems?
- Microcosm experiments : Expose Daphnia magna or Chlorella vulgaris to trace concentrations (0.1–10 ppm) to measure LC and EC.
- Bioaccumulation studies : Use GC-ECD to quantify tissue concentrations in fish (e.g., zebrafish) over 28-day exposure.
- QSAR modeling : Predict persistence and bioaccumulation potential using log (3.8) and solubility (0.2 g/L at 25°C) .
Q. What are the challenges in designing catalytic systems for selective debromination of this compound?
Key issues include:
- Catalyst selectivity : Palladium-based catalysts often over-debrominate to propane, while zinc-copper alloys yield 1,2-dibromopropane.
- Solvent effects : Polar aprotic solvents (e.g., DMF) improve reaction rates but complicate purification. Solution: Optimize bimetallic catalysts (e.g., Ni-Fe) and employ stepwise debromination with in situ NMR monitoring .
Cross-Disciplinary and Mechanistic Questions
Q. How can computational chemistry elucidate reaction mechanisms in this compound’s degradation?
- DFT calculations : Simulate transition states for dehalogenase-catalyzed cleavage of C-Br bonds.
- Molecular docking : Model enzyme-substrate interactions (e.g., haloalkane dehalogenase with this compound) to identify active-site residues.
- Kinetic modeling : Predict degradation rates under varying environmental conditions .
Q. What strategies resolve contradictions between experimental and theoretical data on this compound’s thermodynamic properties?
Discrepancies in enthalpy of formation () often stem from:
- Experimental errors : Calorimetry vs. computational group-additivity methods.
- Conformational isomers : Rotamers affecting gas-phase measurements. Resolution: Use high-purity samples and hybrid DFT-ML approaches to refine predictions .
Q. How can cross-disciplinary approaches improve bioremediation strategies for this compound-contaminated sites?
Integrate:
- Microbial consortia : Combine Agrobacterium radiobacter (dehalogenation) with Pseudomonas spp. (epoxide metabolism).
- Electrochemical sensors : Deploy boron-doped diamond electrodes to monitor degradation in real time.
- Geospatial analysis : Map contamination hotspots using GIS and prioritize remediation zones .
Properties
IUPAC Name |
1,2,3-tribromopropane | |
---|---|---|
Source | PubChem | |
URL | https://pubchem.ncbi.nlm.nih.gov | |
Description | Data deposited in or computed by PubChem | |
InChI |
InChI=1S/C3H5Br3/c4-1-3(6)2-5/h3H,1-2H2 | |
Source | PubChem | |
URL | https://pubchem.ncbi.nlm.nih.gov | |
Description | Data deposited in or computed by PubChem | |
InChI Key |
FHCLGDLYRUPKAM-UHFFFAOYSA-N | |
Source | PubChem | |
URL | https://pubchem.ncbi.nlm.nih.gov | |
Description | Data deposited in or computed by PubChem | |
Canonical SMILES |
C(C(CBr)Br)Br | |
Source | PubChem | |
URL | https://pubchem.ncbi.nlm.nih.gov | |
Description | Data deposited in or computed by PubChem | |
Molecular Formula |
C3H5Br3 | |
Source | PubChem | |
URL | https://pubchem.ncbi.nlm.nih.gov | |
Description | Data deposited in or computed by PubChem | |
DSSTOX Substance ID |
DTXSID9059129 | |
Record name | Propane, 1,2,3-tribromo- | |
Source | EPA DSSTox | |
URL | https://comptox.epa.gov/dashboard/DTXSID9059129 | |
Description | DSSTox provides a high quality public chemistry resource for supporting improved predictive toxicology. | |
Molecular Weight |
280.78 g/mol | |
Source | PubChem | |
URL | https://pubchem.ncbi.nlm.nih.gov | |
Description | Data deposited in or computed by PubChem | |
Physical Description |
Liquid; mp = 16.5 deg C; [Merck Index] Light yellow liquid; mp = 16-17 deg C; [Sigma-Aldrich MSDS] | |
Record name | 1,2,3-Tribromopropane | |
Source | Haz-Map, Information on Hazardous Chemicals and Occupational Diseases | |
URL | https://haz-map.com/Agents/19188 | |
Description | Haz-Map® is an occupational health database designed for health and safety professionals and for consumers seeking information about the adverse effects of workplace exposures to chemical and biological agents. | |
Explanation | Copyright (c) 2022 Haz-Map(R). All rights reserved. Unless otherwise indicated, all materials from Haz-Map are copyrighted by Haz-Map(R). No part of these materials, either text or image may be used for any purpose other than for personal use. Therefore, reproduction, modification, storage in a retrieval system or retransmission, in any form or by any means, electronic, mechanical or otherwise, for reasons other than personal use, is strictly prohibited without prior written permission. | |
CAS No. |
96-11-7 | |
Record name | 1,2,3-Tribromopropane | |
Source | CAS Common Chemistry | |
URL | https://commonchemistry.cas.org/detail?cas_rn=96-11-7 | |
Description | CAS Common Chemistry is an open community resource for accessing chemical information. Nearly 500,000 chemical substances from CAS REGISTRY cover areas of community interest, including common and frequently regulated chemicals, and those relevant to high school and undergraduate chemistry classes. This chemical information, curated by our expert scientists, is provided in alignment with our mission as a division of the American Chemical Society. | |
Explanation | The data from CAS Common Chemistry is provided under a CC-BY-NC 4.0 license, unless otherwise stated. | |
Record name | 1,2,3-Tribromopropane | |
Source | ChemIDplus | |
URL | https://pubchem.ncbi.nlm.nih.gov/substance/?source=chemidplus&sourceid=0000096117 | |
Description | ChemIDplus is a free, web search system that provides access to the structure and nomenclature authority files used for the identification of chemical substances cited in National Library of Medicine (NLM) databases, including the TOXNET system. | |
Record name | s-Tribromopropane | |
Source | DTP/NCI | |
URL | https://dtp.cancer.gov/dtpstandard/servlet/dwindex?searchtype=NSC&outputformat=html&searchlist=78932 | |
Description | The NCI Development Therapeutics Program (DTP) provides services and resources to the academic and private-sector research communities worldwide to facilitate the discovery and development of new cancer therapeutic agents. | |
Explanation | Unless otherwise indicated, all text within NCI products is free of copyright and may be reused without our permission. Credit the National Cancer Institute as the source. | |
Record name | Propane, 1,2,3-tribromo- | |
Source | EPA Chemicals under the TSCA | |
URL | https://www.epa.gov/chemicals-under-tsca | |
Description | EPA Chemicals under the Toxic Substances Control Act (TSCA) collection contains information on chemicals and their regulations under TSCA, including non-confidential content from the TSCA Chemical Substance Inventory and Chemical Data Reporting. | |
Record name | Propane, 1,2,3-tribromo- | |
Source | EPA DSSTox | |
URL | https://comptox.epa.gov/dashboard/DTXSID9059129 | |
Description | DSSTox provides a high quality public chemistry resource for supporting improved predictive toxicology. | |
Record name | 1,2,3-tribromopropane | |
Source | European Chemicals Agency (ECHA) | |
URL | https://echa.europa.eu/substance-information/-/substanceinfo/100.002.254 | |
Description | The European Chemicals Agency (ECHA) is an agency of the European Union which is the driving force among regulatory authorities in implementing the EU's groundbreaking chemicals legislation for the benefit of human health and the environment as well as for innovation and competitiveness. | |
Explanation | Use of the information, documents and data from the ECHA website is subject to the terms and conditions of this Legal Notice, and subject to other binding limitations provided for under applicable law, the information, documents and data made available on the ECHA website may be reproduced, distributed and/or used, totally or in part, for non-commercial purposes provided that ECHA is acknowledged as the source: "Source: European Chemicals Agency, http://echa.europa.eu/". Such acknowledgement must be included in each copy of the material. ECHA permits and encourages organisations and individuals to create links to the ECHA website under the following cumulative conditions: Links can only be made to webpages that provide a link to the Legal Notice page. | |
Record name | 1,2,3-TRIBROMOPROPANE | |
Source | FDA Global Substance Registration System (GSRS) | |
URL | https://gsrs.ncats.nih.gov/ginas/app/beta/substances/D2R8L96TOV | |
Description | The FDA Global Substance Registration System (GSRS) enables the efficient and accurate exchange of information on what substances are in regulated products. Instead of relying on names, which vary across regulatory domains, countries, and regions, the GSRS knowledge base makes it possible for substances to be defined by standardized, scientific descriptions. | |
Explanation | Unless otherwise noted, the contents of the FDA website (www.fda.gov), both text and graphics, are not copyrighted. They are in the public domain and may be republished, reprinted and otherwise used freely by anyone without the need to obtain permission from FDA. Credit to the U.S. Food and Drug Administration as the source is appreciated but not required. | |
Retrosynthesis Analysis
AI-Powered Synthesis Planning: Our tool employs the Template_relevance Pistachio, Template_relevance Bkms_metabolic, Template_relevance Pistachio_ringbreaker, Template_relevance Reaxys, Template_relevance Reaxys_biocatalysis model, leveraging a vast database of chemical reactions to predict feasible synthetic routes.
One-Step Synthesis Focus: Specifically designed for one-step synthesis, it provides concise and direct routes for your target compounds, streamlining the synthesis process.
Accurate Predictions: Utilizing the extensive PISTACHIO, BKMS_METABOLIC, PISTACHIO_RINGBREAKER, REAXYS, REAXYS_BIOCATALYSIS database, our tool offers high-accuracy predictions, reflecting the latest in chemical research and data.
Strategy Settings
Precursor scoring | Relevance Heuristic |
---|---|
Min. plausibility | 0.01 |
Model | Template_relevance |
Template Set | Pistachio/Bkms_metabolic/Pistachio_ringbreaker/Reaxys/Reaxys_biocatalysis |
Top-N result to add to graph | 6 |
Feasible Synthetic Routes
Disclaimer and Information on In-Vitro Research Products
Please be aware that all articles and product information presented on BenchChem are intended solely for informational purposes. The products available for purchase on BenchChem are specifically designed for in-vitro studies, which are conducted outside of living organisms. In-vitro studies, derived from the Latin term "in glass," involve experiments performed in controlled laboratory settings using cells or tissues. It is important to note that these products are not categorized as medicines or drugs, and they have not received approval from the FDA for the prevention, treatment, or cure of any medical condition, ailment, or disease. We must emphasize that any form of bodily introduction of these products into humans or animals is strictly prohibited by law. It is essential to adhere to these guidelines to ensure compliance with legal and ethical standards in research and experimentation.