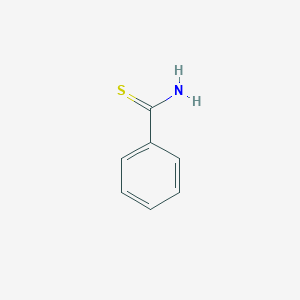
Thiobenzamide
Overview
Description
Thiobenzamide is a chemical compound with the linear formula C6H5CSNH2 . It is used in the preparation of amide and amidine adducts . It also plays a role in the synthesis of 4-oxo-4H-chromene-3-carbothioic acid N-phenylamides .
Synthesis Analysis
Thiobenzamide has been synthesized and characterized by various methods . One method involves the copolymerization of L-lactide and a lactide functionalized with 4-hydroxythiobenzamide . Another method involves the reaction of cyanobenzene and thioacetamide .
Molecular Structure Analysis
The crystal and molecular structure of Thiobenzamide has been determined by single crystal X-ray diffraction analysis . The molecular arrangement in the crystal structure of Thiobenzamide can be described on the basis of supramolecular dimeric synthons built up by four independent Thiobenzamide molecules stacked via N-H…S hydrogen bonds .
Chemical Reactions Analysis
Thiobenzamide has been used in the synthesis of various compounds . For instance, it has been used in the synthesis of a Cd (II) complex, dichlorothiobenzamidecadmium (II) [Cd (TBA)Cl 2], which has been characterized by FT-IR, FT-Raman and UV–Vis spectroscopy .
Physical And Chemical Properties Analysis
Thiobenzamide has a molecular weight of 137.20 and a linear formula of C6H5CSNH2 . It has a melting point of 113-117 °C .
Scientific Research Applications
Preparation of Amide and Amidine Adducts
Thiobenzamide is utilized in the synthesis of amide and amidine adducts, which are important intermediates in organic chemistry for further chemical transformations .
Synthesis of 4-Oxo-4H-Chromene-3-Carbothioic Acid N-Phenylamides
It serves as a precursor in the preparation of N-phenylamides derivatives, which are compounds of interest due to their potential pharmacological properties .
Raw Material in Organic Synthesis
As a raw material, Thiobenzamide is involved in various organic synthesis processes, contributing to the creation of diverse organic compounds .
Lipoxygenase Catalyzed Reactions
In biochemical research, Thiobenzamide has been studied for its role in lipoxygenase catalyzed reactions, particularly in the metabolism of xenobiotics .
Structural Analysis in Chemistry
Thiobenzamide derivatives have been analyzed using X-ray structure analyses to understand deviations from planarity in molecular structures, which is significant for the design of new molecules with desired properties .
Synthesis of Thioamides
It is also used in the eco-friendly synthesis of thioamides from aryl nitriles, which are valuable building blocks in pharmaceutical chemistry and drug design due to their ability to mimic amide functions in biomolecules .
Each application showcases the versatility and importance of Thiobenzamide in scientific research across various fields of chemistry.
Thermo Fisher Scientific - Thiobenzamide Applications Springer - Sterically crowded thioamides MilliporeSigma - Thiobenzamide MDPI - Towards More Practical Methods for the Synthesis of Thioamides RSC Publishing - Efficient Protocol for the Synthesis of Thioamides
Mechanism of Action
Target of Action
Thiobenzamide primarily targets the D2 receptor . This receptor plays a crucial role in the chemoreceptor trigger zone (CTZ) of the medulla oblongata . Thiobenzamide derivatives have also been used as novel pesticides, acting as chitin synthesis inhibitors .
Mode of Action
Thiobenzamide acts as an antagonist of the D2 receptor . It is believed to affect the chemoreceptor trigger zone (CTZ) of the medulla oblongata, which helps suppress nausea and vomiting .
Biochemical Pathways
It is known that thiobenzamide is metabolized via sequential oxygenations of the thioamide sulfur atom by flavoprotein monooxygenases or cytochromes p450 . This activity results in the elimination of the thioamide sulfur and formation of nitrile and/or amide derivatives .
Pharmacokinetics
It is known that the bioavailability of related compounds can be influenced by factors such as the method of administration and the presence of other substances .
Result of Action
The molecular and cellular effects of thiobenzamide’s action are largely dependent on its interaction with the D2 receptor. By antagonizing this receptor, thiobenzamide can suppress nausea and vomiting . Additionally, thiobenzamide derivatives have shown insecticidal activity, indicating potential utility in pest control .
Action Environment
The action, efficacy, and stability of thiobenzamide can be influenced by various environmental factors. For instance, the presence of other substances can affect its bioavailability and metabolism . More research is needed to fully understand how environmental factors influence the action of thiobenzamide.
Safety and Hazards
Future Directions
Thiobenzamide and its derivatives have a wide range of applications due to their pharmacological properties . They are important in the pharmaceutical chemistry and drug design, owing to their ability to mimic the amide function in biomolecules while retaining or developing biological activity . Future research may focus on developing more practical methods for the chemical synthesis of thioamides using sulfuration agents .
properties
IUPAC Name |
benzenecarbothioamide | |
---|---|---|
Source | PubChem | |
URL | https://pubchem.ncbi.nlm.nih.gov | |
Description | Data deposited in or computed by PubChem | |
InChI |
InChI=1S/C7H7NS/c8-7(9)6-4-2-1-3-5-6/h1-5H,(H2,8,9) | |
Source | PubChem | |
URL | https://pubchem.ncbi.nlm.nih.gov | |
Description | Data deposited in or computed by PubChem | |
InChI Key |
QIOZLISABUUKJY-UHFFFAOYSA-N | |
Source | PubChem | |
URL | https://pubchem.ncbi.nlm.nih.gov | |
Description | Data deposited in or computed by PubChem | |
Canonical SMILES |
C1=CC=C(C=C1)C(=S)N | |
Source | PubChem | |
URL | https://pubchem.ncbi.nlm.nih.gov | |
Description | Data deposited in or computed by PubChem | |
Molecular Formula |
C7H7NS | |
Source | PubChem | |
URL | https://pubchem.ncbi.nlm.nih.gov | |
Description | Data deposited in or computed by PubChem | |
DSSTOX Substance ID |
DTXSID8062280 | |
Record name | Benzenecarbothioamide | |
Source | EPA DSSTox | |
URL | https://comptox.epa.gov/dashboard/DTXSID8062280 | |
Description | DSSTox provides a high quality public chemistry resource for supporting improved predictive toxicology. | |
Molecular Weight |
137.20 g/mol | |
Source | PubChem | |
URL | https://pubchem.ncbi.nlm.nih.gov | |
Description | Data deposited in or computed by PubChem | |
Product Name |
Thiobenzamide | |
CAS RN |
2227-79-4 | |
Record name | Thiobenzamide | |
Source | CAS Common Chemistry | |
URL | https://commonchemistry.cas.org/detail?cas_rn=2227-79-4 | |
Description | CAS Common Chemistry is an open community resource for accessing chemical information. Nearly 500,000 chemical substances from CAS REGISTRY cover areas of community interest, including common and frequently regulated chemicals, and those relevant to high school and undergraduate chemistry classes. This chemical information, curated by our expert scientists, is provided in alignment with our mission as a division of the American Chemical Society. | |
Explanation | The data from CAS Common Chemistry is provided under a CC-BY-NC 4.0 license, unless otherwise stated. | |
Record name | Thiobenzamide | |
Source | ChemIDplus | |
URL | https://pubchem.ncbi.nlm.nih.gov/substance/?source=chemidplus&sourceid=0002227794 | |
Description | ChemIDplus is a free, web search system that provides access to the structure and nomenclature authority files used for the identification of chemical substances cited in National Library of Medicine (NLM) databases, including the TOXNET system. | |
Record name | Benzenecarbothioamide | |
Source | EPA Chemicals under the TSCA | |
URL | https://www.epa.gov/chemicals-under-tsca | |
Description | EPA Chemicals under the Toxic Substances Control Act (TSCA) collection contains information on chemicals and their regulations under TSCA, including non-confidential content from the TSCA Chemical Substance Inventory and Chemical Data Reporting. | |
Record name | Benzenecarbothioamide | |
Source | EPA DSSTox | |
URL | https://comptox.epa.gov/dashboard/DTXSID8062280 | |
Description | DSSTox provides a high quality public chemistry resource for supporting improved predictive toxicology. | |
Record name | Thiobenzamide | |
Source | European Chemicals Agency (ECHA) | |
URL | https://echa.europa.eu/substance-information/-/substanceinfo/100.017.060 | |
Description | The European Chemicals Agency (ECHA) is an agency of the European Union which is the driving force among regulatory authorities in implementing the EU's groundbreaking chemicals legislation for the benefit of human health and the environment as well as for innovation and competitiveness. | |
Explanation | Use of the information, documents and data from the ECHA website is subject to the terms and conditions of this Legal Notice, and subject to other binding limitations provided for under applicable law, the information, documents and data made available on the ECHA website may be reproduced, distributed and/or used, totally or in part, for non-commercial purposes provided that ECHA is acknowledged as the source: "Source: European Chemicals Agency, http://echa.europa.eu/". Such acknowledgement must be included in each copy of the material. ECHA permits and encourages organisations and individuals to create links to the ECHA website under the following cumulative conditions: Links can only be made to webpages that provide a link to the Legal Notice page. | |
Record name | THIOBENZAMIDE | |
Source | FDA Global Substance Registration System (GSRS) | |
URL | https://gsrs.ncats.nih.gov/ginas/app/beta/substances/8799VM9SXC | |
Description | The FDA Global Substance Registration System (GSRS) enables the efficient and accurate exchange of information on what substances are in regulated products. Instead of relying on names, which vary across regulatory domains, countries, and regions, the GSRS knowledge base makes it possible for substances to be defined by standardized, scientific descriptions. | |
Explanation | Unless otherwise noted, the contents of the FDA website (www.fda.gov), both text and graphics, are not copyrighted. They are in the public domain and may be republished, reprinted and otherwise used freely by anyone without the need to obtain permission from FDA. Credit to the U.S. Food and Drug Administration as the source is appreciated but not required. | |
Synthesis routes and methods
Procedure details
Retrosynthesis Analysis
AI-Powered Synthesis Planning: Our tool employs the Template_relevance Pistachio, Template_relevance Bkms_metabolic, Template_relevance Pistachio_ringbreaker, Template_relevance Reaxys, Template_relevance Reaxys_biocatalysis model, leveraging a vast database of chemical reactions to predict feasible synthetic routes.
One-Step Synthesis Focus: Specifically designed for one-step synthesis, it provides concise and direct routes for your target compounds, streamlining the synthesis process.
Accurate Predictions: Utilizing the extensive PISTACHIO, BKMS_METABOLIC, PISTACHIO_RINGBREAKER, REAXYS, REAXYS_BIOCATALYSIS database, our tool offers high-accuracy predictions, reflecting the latest in chemical research and data.
Strategy Settings
Precursor scoring | Relevance Heuristic |
---|---|
Min. plausibility | 0.01 |
Model | Template_relevance |
Template Set | Pistachio/Bkms_metabolic/Pistachio_ringbreaker/Reaxys/Reaxys_biocatalysis |
Top-N result to add to graph | 6 |
Feasible Synthetic Routes
Q & A
Q1: What is the molecular formula and weight of Thiobenzamide?
A1: Thiobenzamide has the molecular formula C₇H₇NS and a molecular weight of 137.21 g/mol.
Q2: What spectroscopic data is available for Thiobenzamide?
A2: Researchers utilize various spectroscopic techniques to characterize Thiobenzamide. These include:* Nuclear Magnetic Resonance (NMR) Spectroscopy (¹H and ¹³C): Provides valuable information about the arrangement of hydrogen and carbon atoms within the molecule, helping determine its structure and conformation. []* Infrared (IR) Spectroscopy: Reveals information about the functional groups present in Thiobenzamide by analyzing its interactions with infrared light. []* Ultraviolet-Visible (UV-Vis) Spectroscopy: Helps study the electronic transitions within the molecule and its behavior when interacting with light in the UV-Vis range. []
Q3: How does Thiobenzamide react with metal ions like mercury(II)?
A3: Thiobenzamide forms complexes with mercury(II) ions in acidic aqueous solutions. The predominant complex is a 2:1 ratio of Thiobenzamide to mercury(II). In the presence of excess mercury(II), these complexes decompose into mercury(II) sulfide and an organic product, primarily benzonitrile. The reaction rate is influenced by factors such as pH and the presence of N-substituents on the Thiobenzamide molecule. []
Q4: Can Thiobenzamide be oxidized, and what are the products?
A4: Yes, Thiobenzamide can be oxidized. Researchers have studied its oxidation using various oxidizing agents:* Iodine: Oxidation with iodine leads to a reaction pattern similar to that observed with thiocarbamides. []* Peroxidases: Enzymes like lactoperoxidase, in the presence of hydrogen peroxide, catalyze the S-oxygenation of Thiobenzamide, resulting in the formation of Thiobenzamide S-oxide. []
Q5: What is the Willgerodt-Kindler reaction, and how is Thiobenzamide synthesized using this method?
A5: The Willgerodt-Kindler reaction offers a prominent route for synthesizing thioamides, including Thiobenzamide derivatives. This reaction typically involves reacting an aldehyde or ketone with sulfur and an amine. Researchers have explored a microwave-assisted approach employing montmorillonite K-10 as a heterogeneous acid catalyst, enabling efficient and eco-friendly synthesis of various phenyl (morpholino) methanethiones derivatives, including a novel Thiobenzamide derivative, 4-(morpholine-4-carbonothioyl)benzoic acid. []
Q6: How is computational chemistry used to study Thiobenzamide?
A6: Computational chemistry plays a significant role in understanding the structure and properties of Thiobenzamide and its derivatives. * QSAR Studies: Researchers have developed Quantitative Structure-Activity Relationship (QSAR) models using molecular descriptors like heat of formation, molecular weight, HOMO/LUMO energies, and topological indices to predict the activity of Thiobenzamide derivatives against various Mycobacterium species. These models provide insights into the relationship between the chemical structure and biological activity, aiding in the design of potentially more effective compounds. [, ]* Electron Density Calculations: Ab initio calculations, such as those using the STO-3G basis set, help determine the electron density distribution within the Thiobenzamide molecule, providing information about its reactivity and interactions with other molecules. []
Q7: Are there any insecticidal applications of Thiobenzamide and its derivatives?
A7: Yes, research indicates that Thiobenzamide derivatives show potential as insecticides. Studies on insects like the cotton leafworm (Spodoptera littoralis) demonstrate that certain Thiobenzamide derivatives, particularly those containing halogen and methoxy substituents, exhibit significant insecticidal activity. These compounds are believed to act as insect growth regulators, disrupting chitin synthesis, which is crucial for insect development. [, ]
Q8: How does Thiobenzamide interact with the flavin-containing monooxygenase (FMO) enzyme system?
A8: Thiobenzamide is a substrate for the FMO enzyme system, specifically undergoing S-oxidation to Thiobenzamide S-oxide. This metabolic pathway has been investigated in various systems, including rat liver microsomes and perfused rat liver, highlighting its role in xenobiotic metabolism. Researchers use Thiobenzamide as a probe to study FMO activity and its involvement in drug metabolism. [, ]
Q9: What are the known toxicological effects of Thiobenzamide?
A9: Thiobenzamide is primarily known for its hepatotoxic properties, causing liver damage in animal models. Studies in rats have shown that Thiobenzamide administration leads to: * Liver Damage: Histological examination reveals significant alterations in liver structure following Thiobenzamide exposure. [] * Lipid Peroxidation: Increased levels of conjugated dienes and malondialdehyde in liver microsomes suggest that Thiobenzamide induces oxidative stress and lipid peroxidation, contributing to its toxic effects. []* Thymus Involution: Studies indicate that Thiobenzamide can cause a decrease in thymus weight, particularly in the cortex region, suggesting an impact on the immune system. []
Q10: How does Thiobenzamide's toxicity compare to its metabolites?
A10: Studies comparing the toxicity of Thiobenzamide with its metabolites, Thiobenzamide S-oxide (TBSO) and benzamide (BA), reveal interesting insights:* TBSO: This proximal metabolite shares the thymus-involuting effects of Thiobenzamide, indicating that it might contribute to the overall toxicity profile. []* BA: This terminal metabolite does not appear to cause significant thymus involution, suggesting that it might be less toxic than the parent compound and TBSO. []
Disclaimer and Information on In-Vitro Research Products
Please be aware that all articles and product information presented on BenchChem are intended solely for informational purposes. The products available for purchase on BenchChem are specifically designed for in-vitro studies, which are conducted outside of living organisms. In-vitro studies, derived from the Latin term "in glass," involve experiments performed in controlled laboratory settings using cells or tissues. It is important to note that these products are not categorized as medicines or drugs, and they have not received approval from the FDA for the prevention, treatment, or cure of any medical condition, ailment, or disease. We must emphasize that any form of bodily introduction of these products into humans or animals is strictly prohibited by law. It is essential to adhere to these guidelines to ensure compliance with legal and ethical standards in research and experimentation.