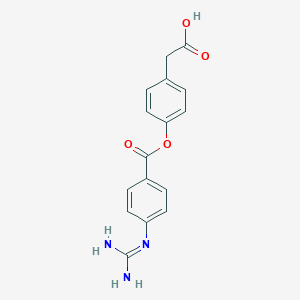
4-(4-Guanidinobenzoyloxy)phenylacetate
Overview
Description
4-(4-Guanidinobenzoyloxy)phenylacetate, also known as this compound, is a useful research compound. Its molecular formula is C16H15N3O4 and its molecular weight is 313.31 g/mol. The purity is usually 95%.
The exact mass of the compound this compound is unknown and the complexity rating of the compound is unknown. Its Medical Subject Headings (MeSH) category is Chemicals and Drugs Category - Organic Chemicals - Amidines - Guanidines - Supplementary Records. The storage condition is unknown. Please store according to label instructions upon receipt of goods.
BenchChem offers high-quality this compound suitable for many research applications. Different packaging options are available to accommodate customers' requirements. Please inquire for more information about this compound including the price, delivery time, and more detailed information at info@benchchem.com.
Mechanism of Action
Target of Action
The primary target of 4-(4-Guanidinobenzoyloxy)phenylacetate, also known as 4-[[4-[(aminoiminomethyl)amino]benzoyl]oxy]benzeneacetic acid, is the Type II Transmembrane Serine Protease (TMPRSS2) . TMPRSS2 plays a crucial role in facilitating the entry of certain viruses, such as SARS-CoV-2, into host cells .
Mode of Action
The compound interacts with its target, TMPRSS2, by inhibiting its protease activity . This interaction prevents TMPRSS2 from cleaving the S1/S2 interface of the viral spike protein, a necessary step for the virus to enter the host cell . The compound’s guanidino moiety forms a salt bridge with D435 in the primary recognition pocket A of TMPRSS2, stabilizing substrate binding .
Biochemical Pathways
The compound is part of a biochemical pathway involving the hydrolysis of camostat mesylate by carboxylesterase (CES) to form this compound . This active metabolite is further hydrolyzed by arylesterase to 4-guanidinobenzoic acid .
Result of Action
The inhibition of TMPRSS2 by this compound prevents the entry of SARS-CoV-2 into host cells . This action could potentially be used to treat COVID-19 .
Action Environment
The action of this compound can be influenced by environmental factors such as the timing of administration relative to meals . For example, the exposure of the compound is significantly lower when administered after a meal or 30 minutes before a meal compared to the fasted state .
Biochemical Analysis
Biochemical Properties
The biochemical properties of 4-(4-Guanidinobenzoyloxy)phenylacetate are primarily related to its interactions with the type II transmembrane serine protease TMPRSS2 . This compound is shown to stabilize through salt bridge formation between the shared guanidino pharmacophore and D435 in pocket A .
Cellular Effects
It is known that this compound plays a role in the entry of coronaviruses, such as SARS-CoV-2, into host cells by cleaving the S1/S2 interface of the viral spike protein .
Molecular Mechanism
The molecular mechanism of this compound involves its interactions with the TMPRSS2 enzyme. It is suggested that residues K342 and W461 are potential contacts involved in TMPRSS2 selective binding and activity .
Metabolic Pathways
Properties
IUPAC Name |
2-[4-[4-(diaminomethylideneamino)benzoyl]oxyphenyl]acetic acid | |
---|---|---|
Source | PubChem | |
URL | https://pubchem.ncbi.nlm.nih.gov | |
Description | Data deposited in or computed by PubChem | |
InChI |
InChI=1S/C16H15N3O4/c17-16(18)19-12-5-3-11(4-6-12)15(22)23-13-7-1-10(2-8-13)9-14(20)21/h1-8H,9H2,(H,20,21)(H4,17,18,19) | |
Source | PubChem | |
URL | https://pubchem.ncbi.nlm.nih.gov | |
Description | Data deposited in or computed by PubChem | |
InChI Key |
XTKGXPFBKPYFDW-UHFFFAOYSA-N | |
Source | PubChem | |
URL | https://pubchem.ncbi.nlm.nih.gov | |
Description | Data deposited in or computed by PubChem | |
Canonical SMILES |
C1=CC(=CC=C1CC(=O)O)OC(=O)C2=CC=C(C=C2)N=C(N)N | |
Source | PubChem | |
URL | https://pubchem.ncbi.nlm.nih.gov | |
Description | Data deposited in or computed by PubChem | |
Molecular Formula |
C16H15N3O4 | |
Source | PubChem | |
URL | https://pubchem.ncbi.nlm.nih.gov | |
Description | Data deposited in or computed by PubChem | |
Molecular Weight |
313.31 g/mol | |
Source | PubChem | |
URL | https://pubchem.ncbi.nlm.nih.gov | |
Description | Data deposited in or computed by PubChem | |
Retrosynthesis Analysis
AI-Powered Synthesis Planning: Our tool employs the Template_relevance Pistachio, Template_relevance Bkms_metabolic, Template_relevance Pistachio_ringbreaker, Template_relevance Reaxys, Template_relevance Reaxys_biocatalysis model, leveraging a vast database of chemical reactions to predict feasible synthetic routes.
One-Step Synthesis Focus: Specifically designed for one-step synthesis, it provides concise and direct routes for your target compounds, streamlining the synthesis process.
Accurate Predictions: Utilizing the extensive PISTACHIO, BKMS_METABOLIC, PISTACHIO_RINGBREAKER, REAXYS, REAXYS_BIOCATALYSIS database, our tool offers high-accuracy predictions, reflecting the latest in chemical research and data.
Strategy Settings
Precursor scoring | Relevance Heuristic |
---|---|
Min. plausibility | 0.01 |
Model | Template_relevance |
Template Set | Pistachio/Bkms_metabolic/Pistachio_ringbreaker/Reaxys/Reaxys_biocatalysis |
Top-N result to add to graph | 6 |
Feasible Synthetic Routes
Q1: How does 4-(4-Guanidinobenzoyloxy)phenylacetate exert its primary effect?
A1: this compound, often investigated as its methanesulfonate salt (e.g., FOY-305), acts primarily as a protease inhibitor. [, , ] While it exhibits a broad spectrum of inhibition, it shows notable activity against trypsin, a key digestive enzyme. [, ] This trypsin-inhibiting capacity is central to many of its observed effects, including its potential influence on pancreatic function and tumor growth.
Q2: The research mentions an influence on cholecystokinin-pancreozymin (CCK). Can you elaborate on this relationship?
A2: Studies using isolated rat duodenum models demonstrated that introducing various trypsin inhibitors, including this compound derivatives, into the duodenum led to increased levels of biologically active CCK in the surrounding blood vessels. [] This finding suggests a potential link between trypsin inhibition and CCK release, although the exact mechanism remains unclear.
Q3: Could you explain how this compound might impact tumor growth?
A3: Research using mouse models has explored the potential antitumor effects of this compound methanesulfonate. [, , , ] In studies involving 3-methylcholanthrene-induced carcinomas, both oral and intraperitoneal administration of the compound showed significant inhibition of tumor growth and increased survival times in mice. [, , ] While the precise mechanisms are still under investigation, the inhibition of kinin-forming proteases like trypsin, plasmin, and kallikrein is thought to play a role. []
Q4: Are there any insights into the absorption and distribution of this compound methanesulfonate in the body?
A4: Studies using a rat intestinal loop model showed that this compound methanesulfonate is absorbed most effectively from the rectum, with lower absorption rates observed in various sections of the small intestine. [, ] This difference in absorption is attributed to rapid degradation of the compound by esterase enzymes present in the small intestine. []
Q5: Beyond its potential in cancer research, are there other areas where this compound derivatives have shown promise?
A6: Yes, research suggests that this compound methanesulfonate could be beneficial in treating acute pancreatitis. [] Clinical trials comparing its efficacy to aprotinin (Trasylol) demonstrated promising results, with the compound showing effectiveness and safety profiles comparable to the established treatment. []
Q6: What about the potential for this compound derivatives to inhibit phospholipase A2?
A7: Studies investigating the inhibitory effects of various protease inhibitors, including this compound derivatives, on phospholipase A2 found that a derivative, gabexate mesilate (FOY), exhibited a 50% reduction in enzyme activity at a concentration of 5 x 10-4 mol/L. [] Similarly, camostat (another derivative) achieved the same level of inhibition at a concentration of 10-3 mol/L. [] This finding suggests potential applications for these compounds in conditions where modulating phospholipase A2 activity is desirable.
Q7: Has any research explored using this compound derivatives to enhance the delivery of other therapeutic agents?
A8: Yes, recent research explored using a modified trimethyl chitosan nanoparticle conjugated with a this compound derivative (FOY-251) and a goblet cell-targeting peptide (CSK) for oral insulin delivery. [] The study demonstrated the potential of this approach to improve insulin stability against trypsin degradation, indicating a promising strategy for enhancing oral bioavailability of peptide drugs. []
Q8: What are the next steps in research on this compound and its derivatives?
A8: Future research should focus on:
- Structure-activity relationship studies: Investigating how structural modifications to this compound influence its activity, potency, and selectivity could lead to more effective and targeted therapies. []
- Improving bioavailability and stability: Overcoming limitations like rapid degradation in the digestive tract is key for realizing the full therapeutic potential of these compounds. [, ]
Disclaimer and Information on In-Vitro Research Products
Please be aware that all articles and product information presented on BenchChem are intended solely for informational purposes. The products available for purchase on BenchChem are specifically designed for in-vitro studies, which are conducted outside of living organisms. In-vitro studies, derived from the Latin term "in glass," involve experiments performed in controlled laboratory settings using cells or tissues. It is important to note that these products are not categorized as medicines or drugs, and they have not received approval from the FDA for the prevention, treatment, or cure of any medical condition, ailment, or disease. We must emphasize that any form of bodily introduction of these products into humans or animals is strictly prohibited by law. It is essential to adhere to these guidelines to ensure compliance with legal and ethical standards in research and experimentation.