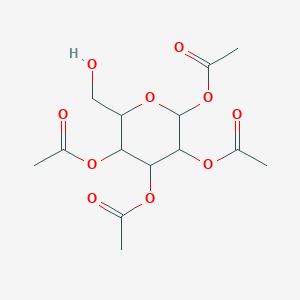
1,2,3,4-Tetra-O-acetyl-beta-D-glucopyranose
Overview
Description
1,2,3,4-Tetra-O-acetyl-beta-D-glucopyranose is a carbohydrate derivative commonly used in organic synthesis. It is a protected form of D-glucose, where the hydroxyl groups are acetylated. This compound is often utilized in the synthesis of disaccharides and D-glucose-6-phosphate .
Biochemical Analysis
Biochemical Properties
1,2,3,4-Tetra-O-acetyl-beta-D-glucopyranose plays a crucial role in biochemical reactions, particularly in the synthesis of glycosides, oligosaccharides, and glycoconjugates. The compound interacts with various enzymes, such as glycosyltransferases and glycosidases, which are involved in the formation and hydrolysis of glycosidic bonds. These interactions are essential for studying glycosylation mechanisms and the stereochemistry of glycosidic bonds . Additionally, phosphorylated derivatives of this compound have been valuable in the study of substrates for inositol synthase .
Cellular Effects
This compound influences various cellular processes, including cell signaling pathways, gene expression, and cellular metabolism. The compound’s role in glycosylation mechanisms affects the formation of glycoproteins and glycolipids, which are crucial for cell signaling and communication. By modulating the activity of glycosyltransferases and glycosidases, this compound can impact the expression of genes involved in carbohydrate metabolism and biosynthesis .
Molecular Mechanism
At the molecular level, this compound exerts its effects through binding interactions with enzymes and other biomolecules. The acetyl groups on the glucose molecule protect the hydroxyl groups during synthetic reactions, allowing for selective deprotection and further functionalization. This protection is crucial for studying the specificity and kinetics of glycosyltransferases and glycosidases, providing insights into carbohydrate metabolism and biosynthesis . Additionally, the compound’s role in the synthesis of disaccharides and D-glucose-6-phosphate highlights its importance in biochemical pathways .
Temporal Effects in Laboratory Settings
In laboratory settings, the effects of this compound can change over time due to its stability and degradation. The compound is stable under standard storage conditions, but its acetyl groups can be hydrolyzed over time, leading to the formation of beta-D-glucopyranose. Long-term studies have shown that the compound’s stability is crucial for its effectiveness in synthetic reactions and enzymatic assays .
Dosage Effects in Animal Models
The effects of this compound vary with different dosages in animal models. At low doses, the compound is generally well-tolerated and does not exhibit significant toxic effects. At high doses, it can cause adverse effects, including toxicity and disruption of cellular functions. Studies have shown that there is a threshold effect, where the compound’s impact on cellular processes becomes more pronounced at higher concentrations .
Metabolic Pathways
This compound is involved in several metabolic pathways, including the synthesis of glycosides, oligosaccharides, and glycoconjugates. The compound interacts with enzymes such as glycosyltransferases and glycosidases, which are essential for the formation and hydrolysis of glycosidic bonds. These interactions affect metabolic flux and metabolite levels, providing insights into carbohydrate metabolism and biosynthesis .
Transport and Distribution
Within cells and tissues, this compound is transported and distributed through interactions with transporters and binding proteins. These interactions influence the compound’s localization and accumulation, affecting its activity and function. The acetyl groups on the glucose molecule enhance its solubility and stability in organic solvents, facilitating its transport and distribution within cells .
Subcellular Localization
This compound is localized in specific subcellular compartments, where it exerts its activity and function. The compound’s acetyl groups act as targeting signals, directing it to specific organelles and compartments within the cell. Post-translational modifications, such as acetylation, play a crucial role in the compound’s subcellular localization and its interactions with biomolecules .
Preparation Methods
Synthetic Routes and Reaction Conditions
1,2,3,4-Tetra-O-acetyl-beta-D-glucopyranose can be synthesized through the acetylation of D-glucose. The process typically involves the reaction of D-glucose with acetic anhydride in the presence of a catalyst such as pyridine. The reaction is carried out under mild conditions, usually at room temperature, to yield the acetylated product .
Industrial Production Methods
In an industrial setting, the production of this compound follows a similar acetylation process but on a larger scale. The reaction conditions are optimized for higher yields and purity, often involving continuous flow reactors and automated systems to ensure consistent product quality .
Chemical Reactions Analysis
Types of Reactions
1,2,3,4-Tetra-O-acetyl-beta-D-glucopyranose undergoes various chemical reactions, including:
Hydrolysis: The acetyl groups can be hydrolyzed under acidic or basic conditions to yield D-glucose.
Oxidation: The compound can be oxidized to form corresponding carboxylic acids.
Substitution: The acetyl groups can be substituted with other functional groups through nucleophilic substitution reactions.
Common Reagents and Conditions
Hydrolysis: Acidic or basic aqueous solutions.
Oxidation: Oxidizing agents such as potassium permanganate or chromium trioxide.
Substitution: Nucleophiles such as amines or alcohols in the presence of a base.
Major Products Formed
Hydrolysis: D-glucose.
Oxidation: Carboxylic acids.
Substitution: Various substituted glucopyranose derivatives.
Scientific Research Applications
Carbohydrate Chemistry
1,2,3,4-Tetra-O-acetyl-beta-D-glucopyranose is primarily utilized as an intermediate in the synthesis of glycosides and oligosaccharides. Its acetylated form enhances stability and solubility, making it easier to manipulate during chemical reactions. Researchers employ this compound to study carbohydrate structures and their biological functions. Notably, it facilitates the synthesis of disaccharides and other complex carbohydrates essential for understanding metabolic pathways and enzyme interactions .
Pharmaceutical Development
In the pharmaceutical sector, this compound plays a crucial role in drug formulation. Its ability to enhance the solubility and stability of pharmaceutical compounds is vital for effective drug delivery systems. For instance, it has been used in the formulation of glucuronide derivatives for cancer therapies, improving the bioavailability of certain drugs . The compound's properties make it suitable for developing prodrugs that can be activated within the body to release therapeutic agents.
Food Industry
This compound serves as a natural sweetening agent and flavor enhancer in food products. Its application provides a healthier alternative to synthetic sweeteners while maintaining desirable taste profiles. The compound's safety and efficacy in food applications have made it popular among manufacturers seeking natural ingredients .
Biotechnology
In biotechnology, this compound contributes to the production of biopolymers and biofuels. It is involved in various processes aimed at creating sustainable practices within industrial applications. For example, its derivatives are used in the synthesis of polysaccharides that can serve as biodegradable materials or renewable energy sources .
Research and Development
The versatility of this compound extends to laboratory research where it aids in studying enzyme interactions and metabolic pathways. Its role as a substrate in various biochemical assays allows researchers to gain insights into biological processes at the molecular level . The compound is also significant in developing new analytical methods for carbohydrate analysis.
Case Studies
Mechanism of Action
The mechanism of action of 1,2,3,4-Tetra-O-acetyl-beta-D-glucopyranose involves its hydrolysis to release D-glucose, which can then participate in various metabolic pathways. The acetyl groups protect the hydroxyl functionalities, allowing selective reactions to occur at other positions on the glucose molecule .
Comparison with Similar Compounds
Similar Compounds
- 1,2,3,4-Tetra-O-acetyl-alpha-D-glucopyranose
- 2,3,4,6-Tetra-O-acetyl-D-galactopyranose
- 2,3,4,6-Tetra-O-benzyl-D-glucopyranose
Uniqueness
1,2,3,4-Tetra-O-acetyl-beta-D-glucopyranose is unique due to its specific beta-anomeric configuration, which influences its reactivity and interaction with enzymes. This configuration distinguishes it from its alpha-anomer and other acetylated sugars .
Biological Activity
1,2,3,4-Tetra-O-acetyl-beta-D-glucopyranose is a carbohydrate derivative that plays a significant role in organic synthesis and biological applications. As a protected form of D-glucose, this compound has been extensively studied for its biochemical properties and biological activities. This article explores its biological activity, mechanisms of action, and applications in various fields.
Chemical Structure and Properties
This compound is characterized by the acetylation of the hydroxyl groups on the glucose molecule. This modification enhances its stability and reactivity in biochemical reactions.
- Chemical Formula : CHO
- Molecular Weight : 288.25 g/mol
- Melting Point : 126-128 °C
This compound is involved in several biochemical processes:
- Synthesis of Glycosides : It serves as a substrate for glycosyltransferases and glycosidases, facilitating the formation of glycosidic bonds crucial for the synthesis of oligosaccharides and glycoconjugates.
- Cell Signaling : The compound influences cell signaling pathways by modulating glycoprotein and glycolipid synthesis, essential for cellular communication .
The biological activity of this compound is primarily attributed to its hydrolysis to D-glucose. This process allows it to participate in various metabolic pathways:
- Enzyme Interactions : The compound interacts with enzymes such as glycosyltransferases, which are vital for carbohydrate metabolism. The acetyl groups protect hydroxyl functionalities during synthetic reactions, enabling selective deprotection and further functionalization .
- Stability and Degradation : In laboratory settings, the stability of this compound is critical. Over time, the acetyl groups can hydrolyze to yield beta-D-glucopyranose, affecting its efficacy in synthetic and enzymatic assays.
Biological Activity
Research has demonstrated that this compound exhibits various biological activities:
- Antimicrobial Properties : Studies indicate that derivatives of this compound can inhibit fungal growth and enhance plant defense mechanisms against pathogens .
- Potential Drug Applications : The compound has been explored for use in drug delivery systems and as a prodrug due to its ability to modulate the release of active pharmaceutical ingredients .
Case Studies
Several studies highlight the biological activity of this compound:
- Plant-Fungus Interaction : A study revealed that this compound can inhibit fungal growth in vitro and delay lesion development in infected plants by inducing the synthesis of defensive compounds like flavonoids.
- Synthesis of Neoglycotrimers : Research demonstrated the successful synthesis of N-glycoside neoglycotrimers from 2,3,4,6-tetra-O-acetyl-beta-D-glucopyranosyl azide. These compounds exhibited high beta-selectivity and potential therapeutic applications .
Comparative Analysis
The biological activity of this compound can be compared with similar compounds:
Compound | Biological Activity | Unique Features |
---|---|---|
1,2,3,4-Tetra-O-acetyl-alpha-D-glucopyranose | Similar glycosidic bond formation | Alpha-anomeric configuration |
2,3,4,6-Tetra-O-benzyl-D-glucopyranose | Enhanced lipophilicity; potential drug carrier | Benzyl protection increases solubility |
2,3,4,6-Tetra-O-acetyl-D-galactopyranose | Antimicrobial properties | Galactose derivative with different reactivity |
Properties
IUPAC Name |
[4,5,6-triacetyloxy-2-(hydroxymethyl)oxan-3-yl] acetate | |
---|---|---|
Details | Computed by Lexichem TK 2.7.0 (PubChem release 2021.05.07) | |
Source | PubChem | |
URL | https://pubchem.ncbi.nlm.nih.gov | |
Description | Data deposited in or computed by PubChem | |
InChI |
InChI=1S/C14H20O10/c1-6(16)20-11-10(5-15)24-14(23-9(4)19)13(22-8(3)18)12(11)21-7(2)17/h10-15H,5H2,1-4H3 | |
Details | Computed by InChI 1.0.6 (PubChem release 2021.05.07) | |
Source | PubChem | |
URL | https://pubchem.ncbi.nlm.nih.gov | |
Description | Data deposited in or computed by PubChem | |
InChI Key |
FEQXFAYSNRWXDW-UHFFFAOYSA-N | |
Details | Computed by InChI 1.0.6 (PubChem release 2021.05.07) | |
Source | PubChem | |
URL | https://pubchem.ncbi.nlm.nih.gov | |
Description | Data deposited in or computed by PubChem | |
Canonical SMILES |
CC(=O)OC1C(OC(C(C1OC(=O)C)OC(=O)C)OC(=O)C)CO | |
Details | Computed by OEChem 2.3.0 (PubChem release 2021.05.07) | |
Source | PubChem | |
URL | https://pubchem.ncbi.nlm.nih.gov | |
Description | Data deposited in or computed by PubChem | |
Molecular Formula |
C14H20O10 | |
Details | Computed by PubChem 2.1 (PubChem release 2021.05.07) | |
Source | PubChem | |
URL | https://pubchem.ncbi.nlm.nih.gov | |
Description | Data deposited in or computed by PubChem | |
DSSTOX Substance ID |
DTXSID10927032 | |
Record name | 1,2,3,4-Tetra-O-acetylhexopyranose | |
Source | EPA DSSTox | |
URL | https://comptox.epa.gov/dashboard/DTXSID10927032 | |
Description | DSSTox provides a high quality public chemistry resource for supporting improved predictive toxicology. | |
Molecular Weight |
348.30 g/mol | |
Details | Computed by PubChem 2.1 (PubChem release 2021.05.07) | |
Source | PubChem | |
URL | https://pubchem.ncbi.nlm.nih.gov | |
Description | Data deposited in or computed by PubChem | |
CAS No. |
13100-46-4 | |
Record name | NSC409250 | |
Source | DTP/NCI | |
URL | https://dtp.cancer.gov/dtpstandard/servlet/dwindex?searchtype=NSC&outputformat=html&searchlist=409250 | |
Description | The NCI Development Therapeutics Program (DTP) provides services and resources to the academic and private-sector research communities worldwide to facilitate the discovery and development of new cancer therapeutic agents. | |
Explanation | Unless otherwise indicated, all text within NCI products is free of copyright and may be reused without our permission. Credit the National Cancer Institute as the source. | |
Record name | 1,2,3,4-Tetra-O-acetylhexopyranose | |
Source | EPA DSSTox | |
URL | https://comptox.epa.gov/dashboard/DTXSID10927032 | |
Description | DSSTox provides a high quality public chemistry resource for supporting improved predictive toxicology. | |
Retrosynthesis Analysis
AI-Powered Synthesis Planning: Our tool employs the Template_relevance Pistachio, Template_relevance Bkms_metabolic, Template_relevance Pistachio_ringbreaker, Template_relevance Reaxys, Template_relevance Reaxys_biocatalysis model, leveraging a vast database of chemical reactions to predict feasible synthetic routes.
One-Step Synthesis Focus: Specifically designed for one-step synthesis, it provides concise and direct routes for your target compounds, streamlining the synthesis process.
Accurate Predictions: Utilizing the extensive PISTACHIO, BKMS_METABOLIC, PISTACHIO_RINGBREAKER, REAXYS, REAXYS_BIOCATALYSIS database, our tool offers high-accuracy predictions, reflecting the latest in chemical research and data.
Strategy Settings
Precursor scoring | Relevance Heuristic |
---|---|
Min. plausibility | 0.01 |
Model | Template_relevance |
Template Set | Pistachio/Bkms_metabolic/Pistachio_ringbreaker/Reaxys/Reaxys_biocatalysis |
Top-N result to add to graph | 6 |
Feasible Synthetic Routes
Q1: What is the role of 1,2,3,4-Tetra-O-acetyl-beta-D-glucopyranose in plant-fungus interactions?
A1: Research suggests that this compound, as part of the FGA mixture, might play a role in enhancing plant defense mechanisms against fungal pathogens. [] Specifically, it was observed to inhibit fungal growth in vitro and, when applied to plants, delayed lesion development caused by fungal infection. [] This protective effect may be linked to the compound's ability to induce the synthesis of defensive compounds in plants, such as flavonoids and phenolic compounds. []
Q2: Can this compound act as an intermediate in chemical reactions?
A2: Yes, this compound has been identified as an intermediate in the sulfuric acid-catalyzed acetylation of carbohydrates. [] Kinetic studies and observation of intermediates confirmed its role in the reaction pathway, which involves the formation of a 6-sulfate ester followed by its acetolysis to produce glucose pentaacetate. []
Q3: Is there structural information available for this compound?
A3: Yes, the crystal structure of anhydrous this compound has been determined at 292 K. [] The study revealed a corrugated layer arrangement of the molecules, held together by partial connectivity due to disorder in the OH group. []
Q4: Has this compound been used in the synthesis of more complex molecules?
A4: Yes, this compound serves as a valuable building block in carbohydrate chemistry. For instance, it was employed in the synthesis of (1→6)-linked disaccharides, which are important targets for protein modification. [] The compound's versatility stems from its ability to undergo glycosylation reactions, enabling the creation of diverse disaccharide structures. []
Q5: Are there any studies on the potential use of this compound derivatives in medicinal chemistry?
A5: While not directly utilizing this compound, research explored the synthesis and biological activity of ferrocenyl carbohydrate conjugates. [] These conjugates incorporated various monosaccharide derivatives, highlighting the potential of sugar-based molecules in drug development. [] Though not directly studied, this research avenue suggests possibilities for exploring the medicinal chemistry potential of this compound derivatives.
Disclaimer and Information on In-Vitro Research Products
Please be aware that all articles and product information presented on BenchChem are intended solely for informational purposes. The products available for purchase on BenchChem are specifically designed for in-vitro studies, which are conducted outside of living organisms. In-vitro studies, derived from the Latin term "in glass," involve experiments performed in controlled laboratory settings using cells or tissues. It is important to note that these products are not categorized as medicines or drugs, and they have not received approval from the FDA for the prevention, treatment, or cure of any medical condition, ailment, or disease. We must emphasize that any form of bodily introduction of these products into humans or animals is strictly prohibited by law. It is essential to adhere to these guidelines to ensure compliance with legal and ethical standards in research and experimentation.