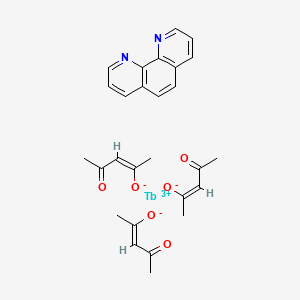
(Z)-4-oxopent-2-en-2-olate;1,10-phenanthroline;terbium(3+)
Overview
Description
(Z)-4-oxopent-2-en-2-olate;1,10-phenanthroline;terbium(3+) is a coordination compound that combines the unique properties of each of its components The compound features a central terbium ion (Tb^3+), which is coordinated by 1,10-phenanthroline and (Z)-4-oxopent-2-en-2-olate ligands
Preparation Methods
Synthetic Routes and Reaction Conditions
The synthesis of (Z)-4-oxopent-2-en-2-olate;1,10-phenanthroline;terbium(3+) typically involves the following steps:
Preparation of (Z)-4-oxopent-2-en-2-olate: This ligand can be synthesized through the aldol condensation of acetylacetone with formaldehyde under basic conditions.
Coordination with Terbium Ion: The terbium ion is introduced by dissolving terbium nitrate in an appropriate solvent, such as ethanol or water.
Addition of 1,10-phenanthroline: 1,10-phenanthroline is added to the solution containing the terbium ion and (Z)-4-oxopent-2-en-2-olate. The mixture is then stirred and heated to facilitate the coordination reaction.
Industrial Production Methods
Industrial production of this compound may involve similar steps but on a larger scale. The reaction conditions, such as temperature, pressure, and solvent choice, are optimized to maximize yield and purity. Techniques such as crystallization and chromatography may be employed to purify the final product.
Chemical Reactions Analysis
Types of Reactions
(Z)-4-oxopent-2-en-2-olate;1,10-phenanthroline;terbium(3+) can undergo various chemical reactions, including:
Oxidation: The compound can be oxidized under specific conditions, leading to changes in the oxidation state of the terbium ion.
Reduction: Reduction reactions can also occur, potentially altering the coordination environment of the terbium ion.
Substitution: Ligand substitution reactions can take place, where one or more ligands are replaced by other coordinating species.
Common Reagents and Conditions
Oxidizing Agents: Hydrogen peroxide, potassium permanganate.
Reducing Agents: Sodium borohydride, lithium aluminum hydride.
Substitution Reagents: Various ligands such as bipyridine, ethylenediamine.
Major Products
The major products of these reactions depend on the specific reagents and conditions used. For example, oxidation may lead to terbium(IV) complexes, while substitution reactions can yield new coordination compounds with different ligands.
Scientific Research Applications
(Z)-4-oxopent-2-en-2-olate;1,10-phenanthroline;terbium(3+) is a coordination compound with applications spanning chemistry, biology, medicine, and industry, owing to its unique coordination environment and luminescent properties. The compound combines a central terbium ion (Tb^3+) coordinated by 1,10-phenanthroline and (Z)-4-oxopent-2-en-2-olate ligands.
Chemistry
(Z)-4-oxopent-2-en-2-olate;1,10-phenanthroline;terbium(3+) serves as a catalyst in diverse organic reactions, facilitating hydrogenation, oxidation, and polymerization due to its unique coordination environment.
Biology
In biological research, the compound is explored as a fluorescent probe because the terbium ion exhibits luminescent properties, making it useful for imaging and sensing applications in biological systems. The biological activity of (Z)-4-oxopent-2-en-2-olate;1,10-phenanthroline;terbium(3+) primarily stems from the coordination of the terbium ion with various biological molecules. The Tb^3+ ion exhibits unique luminescent properties that can be utilized for imaging and sensing applications in biological systems.
Medicine
The compound is investigated for potential use in diagnostic imaging because the luminescent properties of terbium can be harnessed for imaging techniques such as fluorescence microscopy and magnetic resonance imaging (MRI). It can also be used in magnetic resonance imaging (MRI) for enhancing contrast through luminescent probes and in fluorescence-based assays for detecting specific biomolecules or cellular processes.
Industry
(Z)-4-oxopent-2-en-2-olate;1,10-phenanthroline;terbium(3+) is used in the development of advanced materials. Its coordination properties are exploited to create materials with specific electronic, magnetic, and optical properties.
Luminescence Properties
The terbium ion emits characteristic green light upon excitation, useful for fluorescence microscopy for imaging cellular structures and sensing applications, where its luminescence can be modulated by interactions with biomolecules to detect specific biological events.
Antimicrobial Activity
Terbium complexes exhibit enhanced antimicrobial properties compared to their ligands alone. Studies show that Tb^3+ complexes are more effective against bacterial strains such as Staphylococcus aureus and Escherichia coli compared to their respective ligands.
Complex | Microbial Strain | Activity |
---|---|---|
Tb(HDAP)₃·bathophen | Bacillus subtilis | High |
Tb(HDAP)₃·dmph | Candida albicans | Moderate |
Interaction with Proteins
Mechanism of Action
The mechanism of action of (Z)-4-oxopent-2-en-2-olate;1,10-phenanthroline;terbium(3+) involves the interaction of the terbium ion with its ligands. The terbium ion can coordinate with various ligands, altering its electronic structure and properties. This coordination can affect the compound’s reactivity, luminescence, and catalytic activity. The molecular targets and pathways involved depend on the specific application, such as catalysis or imaging.
Comparison with Similar Compounds
Similar Compounds
Terbium(III) acetate: Another terbium coordination compound with different ligands.
Terbium(III) chloride: A simple terbium salt used in various applications.
Terbium(III) nitrate: Commonly used in the synthesis of other terbium complexes.
Uniqueness
(Z)-4-oxopent-2-en-2-olate;1,10-phenanthroline;terbium(3+) is unique due to its specific combination of ligands, which confer distinct properties such as enhanced luminescence and specific catalytic activity. The presence of 1,10-phenanthroline and (Z)-4-oxopent-2-en-2-olate ligands creates a unique coordination environment around the terbium ion, differentiating it from other terbium compounds.
This detailed article provides a comprehensive overview of (Z)-4-oxopent-2-en-2-olate;1,10-phenanthroline;terbium(3+), covering its synthesis, reactions, applications, mechanism of action, and comparison with similar compounds
Biological Activity
(Z)-4-oxopent-2-en-2-olate;1,10-phenanthroline;terbium(3+) is a coordination compound that integrates the unique properties of its components: the terbium ion (Tb^3+), 1,10-phenanthroline, and (Z)-4-oxopent-2-en-2-olate. This compound has garnered significant interest in various fields, particularly in biological research due to its luminescent properties and potential applications in imaging and sensing.
Chemical Composition and Synthesis
The compound is synthesized through a multi-step process involving the coordination of terbium ions with specific ligands. The synthesis typically includes:
- Preparation of (Z)-4-oxopent-2-en-2-olate : This ligand is synthesized via aldol condensation.
- Coordination with Terbium Ion : Terbium nitrate is dissolved in a suitable solvent, followed by the addition of the ligand.
- Incorporation of 1,10-phenanthroline : This ligand enhances the stability and luminescent properties of the complex.
The biological activity of (Z)-4-oxopent-2-en-2-olate;1,10-phenanthroline;terbium(3+) primarily stems from the coordination of the terbium ion with various biological molecules. The Tb^3+ ion exhibits unique luminescent properties that can be utilized for imaging and sensing applications in biological systems.
Luminescence Properties
The terbium ion emits characteristic green light upon excitation, which is attributed to transitions between its energy levels. This luminescence can be harnessed for various applications including:
- Fluorescence Microscopy : The compound can serve as a fluorescent probe for imaging cellular structures.
- Sensing Applications : Its luminescence can be modulated by interactions with biomolecules, making it useful for detecting specific biological events.
Antimicrobial Activity
Research has shown that terbium complexes exhibit enhanced antimicrobial properties compared to their ligands alone. For instance, studies demonstrated that Tb^3+ complexes were more effective against various bacterial strains such as Staphylococcus aureus and Escherichia coli compared to their respective ligands .
Complex | Microbial Strain | Activity |
---|---|---|
Tb(HDAP)₃·bathophen | Bacillus subtilis | High |
Tb(HDAP)₃·dmph | Candida albicans | Moderate |
Interaction with Proteins
The interaction between (Z)-4-oxopent-2-en-2olate;1,10-phenanthroline;terbium(3+) and proteins like DREAM (a calcium-binding protein) has been investigated. It was found that Tb^3+ binding induces conformational changes in DREAM that affect its functionality in signal transduction pathways .
Applications in Medicine
The unique properties of this compound make it a candidate for use in diagnostic imaging techniques. The luminescent properties of terbium can be utilized in:
- Magnetic Resonance Imaging (MRI) : Enhancing contrast through luminescent probes.
- Fluorescence-Based Assays : For detecting specific biomolecules or cellular processes.
Properties
IUPAC Name |
(Z)-4-oxopent-2-en-2-olate;1,10-phenanthroline;terbium(3+) | |
---|---|---|
Source | PubChem | |
URL | https://pubchem.ncbi.nlm.nih.gov | |
Description | Data deposited in or computed by PubChem | |
InChI |
InChI=1S/C12H8N2.3C5H8O2.Tb/c1-3-9-5-6-10-4-2-8-14-12(10)11(9)13-7-1;3*1-4(6)3-5(2)7;/h1-8H;3*3,6H,1-2H3;/q;;;;+3/p-3/b;3*4-3-; | |
Source | PubChem | |
URL | https://pubchem.ncbi.nlm.nih.gov | |
Description | Data deposited in or computed by PubChem | |
InChI Key |
YUDOUPKNKVFAMM-XUHIWKAKSA-K | |
Source | PubChem | |
URL | https://pubchem.ncbi.nlm.nih.gov | |
Description | Data deposited in or computed by PubChem | |
Canonical SMILES |
CC(=CC(=O)C)[O-].CC(=CC(=O)C)[O-].CC(=CC(=O)C)[O-].C1=CC2=C(C3=C(C=CC=N3)C=C2)N=C1.[Tb+3] | |
Source | PubChem | |
URL | https://pubchem.ncbi.nlm.nih.gov | |
Description | Data deposited in or computed by PubChem | |
Isomeric SMILES |
C/C(=C/C(=O)C)/[O-].C/C(=C/C(=O)C)/[O-].C/C(=C/C(=O)C)/[O-].C1=CC2=C(N=C1)C3=C(C=C2)C=CC=N3.[Tb+3] | |
Source | PubChem | |
URL | https://pubchem.ncbi.nlm.nih.gov | |
Description | Data deposited in or computed by PubChem | |
Molecular Formula |
C27H29N2O6Tb | |
Source | PubChem | |
URL | https://pubchem.ncbi.nlm.nih.gov | |
Description | Data deposited in or computed by PubChem | |
Molecular Weight |
636.5 g/mol | |
Source | PubChem | |
URL | https://pubchem.ncbi.nlm.nih.gov | |
Description | Data deposited in or computed by PubChem | |
Retrosynthesis Analysis
AI-Powered Synthesis Planning: Our tool employs the Template_relevance Pistachio, Template_relevance Bkms_metabolic, Template_relevance Pistachio_ringbreaker, Template_relevance Reaxys, Template_relevance Reaxys_biocatalysis model, leveraging a vast database of chemical reactions to predict feasible synthetic routes.
One-Step Synthesis Focus: Specifically designed for one-step synthesis, it provides concise and direct routes for your target compounds, streamlining the synthesis process.
Accurate Predictions: Utilizing the extensive PISTACHIO, BKMS_METABOLIC, PISTACHIO_RINGBREAKER, REAXYS, REAXYS_BIOCATALYSIS database, our tool offers high-accuracy predictions, reflecting the latest in chemical research and data.
Strategy Settings
Precursor scoring | Relevance Heuristic |
---|---|
Min. plausibility | 0.01 |
Model | Template_relevance |
Template Set | Pistachio/Bkms_metabolic/Pistachio_ringbreaker/Reaxys/Reaxys_biocatalysis |
Top-N result to add to graph | 6 |
Feasible Synthetic Routes
Q1: What was the primary research question addressed by the TCOG T3207 study?
A1: The TCOG T3207 study aimed to determine if adding adjuvant CRT to standard adjuvant gemcitabine therapy would improve recurrence-free survival (RFS) in patients with resectable PDAC. [] This was the study's primary endpoint.
Q2: Did the addition of adjuvant CRT improve RFS in the T3207 study? What were the key findings?
A2: The study found that adding CRT did not significantly improve RFS in patients receiving adjuvant gemcitabine. [] While there was a marginal benefit in reducing loco-regional recurrence, this did not translate to a statistically significant improvement in overall RFS. The authors suggest further research is needed, particularly regarding the potential role of CRT alongside newer multi-agent adjuvant therapies like modified FOLFIRINOX. []
Disclaimer and Information on In-Vitro Research Products
Please be aware that all articles and product information presented on BenchChem are intended solely for informational purposes. The products available for purchase on BenchChem are specifically designed for in-vitro studies, which are conducted outside of living organisms. In-vitro studies, derived from the Latin term "in glass," involve experiments performed in controlled laboratory settings using cells or tissues. It is important to note that these products are not categorized as medicines or drugs, and they have not received approval from the FDA for the prevention, treatment, or cure of any medical condition, ailment, or disease. We must emphasize that any form of bodily introduction of these products into humans or animals is strictly prohibited by law. It is essential to adhere to these guidelines to ensure compliance with legal and ethical standards in research and experimentation.