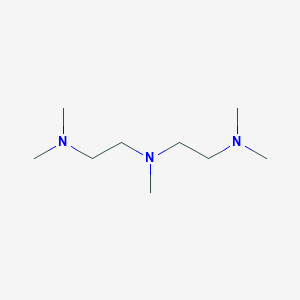
1,1,4,7,7-Pentamethyldiethylenetriamine
Overview
Description
1,1,4,7,7-Pentamethyldiethylenetriamine is an organic compound with the chemical formula C₉H₂₃N₃. It is a colorless liquid that is highly soluble in water and other organic solvents. This compound is known for its use as a tridentate ligand in organolithium chemistry, where it helps to modify the reactivity of organolithium compounds .
Mechanism of Action
Target of Action
1,1,4,7,7-Pentamethyldiethylenetriamine, also known as Pentamethyldiethylenetriamine or PMDTA, is primarily used as a ligand in organolithium chemistry . It is a basic, bulky, and flexible tridentate ligand . The primary targets of PMDTA are organolithium compounds .
Mode of Action
PMDTA interacts with its targets, the organolithium compounds, by binding to the lithium center . This binding enhances the reactivity of organolithium compounds . PMDTA behaves analogously to the di-tertiary amine TMEDA, but since it is tridentate, it binds more strongly to lithium . In contrast to TMEDA, PMDTA forms monomeric complexes with organolithium compounds .
Biochemical Pathways
The biochemical pathways affected by PMDTA primarily involve the reactivity of organolithium compounds . PMDTA modifies the reactivity of these compounds, which deaggregate in the presence of Lewis bases . Both PMDTA and TMEDA affect the regiochemistry of metalation .
Pharmacokinetics
It is known that pmdta is miscible in water , which could potentially influence its absorption, distribution, metabolism, and excretion (ADME) properties.
Result of Action
The result of PMDTA’s action is an enhancement of the reactivity of organolithium compounds . This increased reactivity can be utilized in various chemical reactions, including the synthesis of polymers .
Action Environment
PMDTA is sensitive to air and moisture . It should be stored under inert gas and is incompatible with strong oxidizing agents and strong acids . These environmental factors can influence the action, efficacy, and stability of PMDTA.
Preparation Methods
1,1,4,7,7-Pentamethyldiethylenetriamine is typically synthesized from diethylenetriamine through the Eschweiler-Clarke reaction. This reaction involves the use of formaldehyde and formic acid to methylate the amine groups . The general reaction is as follows: [ \text{(H}_2\text{N[CH}_2\text{]}_2\text{)}_2\text{NH} + 5\text{CH}_2\text{O} + 5\text{HCO}_2\text{H} \rightarrow \text{(Me}_2\text{N[CH}_2\text{]}_2\text{)}_2\text{NMe} + 5\text{CO}_2 + 5\text{H}_2\text{O} ]
For industrial production, the compound can also be synthesized using a high-pressure reactor with a palladium on carbon catalyst. This method involves the hydrogenation of diethylenetriamine in the presence of formaldehyde .
Chemical Reactions Analysis
1,1,4,7,7-Pentamethyldiethylenetriamine undergoes various chemical reactions, including:
Oxidation: It can be oxidized to form corresponding oxides.
Reduction: It can be reduced under specific conditions to form simpler amines.
Substitution: It can undergo substitution reactions where one or more of its hydrogen atoms are replaced by other atoms or groups.
Common reagents used in these reactions include oxidizing agents like hydrogen peroxide, reducing agents like lithium aluminum hydride, and various halogenating agents. The major products formed depend on the specific reaction conditions and reagents used .
Scientific Research Applications
1,1,4,7,7-Pentamethyldiethylenetriamine is widely used in scientific research due to its unique properties:
Chemistry: It is used as a ligand in organolithium chemistry to enhance the reactivity of organolithium compounds.
Biology: It is used in the synthesis of various biologically active compounds.
Medicine: It serves as an intermediate in the synthesis of pharmaceuticals.
Industry: It is used as a catalyst in the production of polyurethane foams and other polymers.
Comparison with Similar Compounds
1,1,4,7,7-Pentamethyldiethylenetriamine is unique due to its tridentate ligand properties. Similar compounds include:
Diethylenetriamine: Unlike this compound, diethylenetriamine has primary and secondary amine groups, making it less sterically hindered.
Triethylenetetramine: This compound has four nitrogen atoms and forms more complex structures with metal ions.
N,N,N’,N’-Tetramethylethylenediamine: This compound is a bidentate ligand and forms less stable complexes compared to this compound.
This compound stands out due to its ability to form highly stable, monomeric complexes with organolithium compounds, which significantly enhances their reactivity .
Properties
IUPAC Name |
N'-[2-(dimethylamino)ethyl]-N,N,N'-trimethylethane-1,2-diamine | |
---|---|---|
Source | PubChem | |
URL | https://pubchem.ncbi.nlm.nih.gov | |
Description | Data deposited in or computed by PubChem | |
InChI |
InChI=1S/C9H23N3/c1-10(2)6-8-12(5)9-7-11(3)4/h6-9H2,1-5H3 | |
Source | PubChem | |
URL | https://pubchem.ncbi.nlm.nih.gov | |
Description | Data deposited in or computed by PubChem | |
InChI Key |
UKODFQOELJFMII-UHFFFAOYSA-N | |
Source | PubChem | |
URL | https://pubchem.ncbi.nlm.nih.gov | |
Description | Data deposited in or computed by PubChem | |
Canonical SMILES |
CN(C)CCN(C)CCN(C)C | |
Source | PubChem | |
URL | https://pubchem.ncbi.nlm.nih.gov | |
Description | Data deposited in or computed by PubChem | |
Molecular Formula |
C9H23N3 | |
Source | PubChem | |
URL | https://pubchem.ncbi.nlm.nih.gov | |
Description | Data deposited in or computed by PubChem | |
DSSTOX Substance ID |
DTXSID7029249 | |
Record name | 1,1,4,7,7-Pentamethyldiethylenetriamine | |
Source | EPA DSSTox | |
URL | https://comptox.epa.gov/dashboard/DTXSID7029249 | |
Description | DSSTox provides a high quality public chemistry resource for supporting improved predictive toxicology. | |
Molecular Weight |
173.30 g/mol | |
Source | PubChem | |
URL | https://pubchem.ncbi.nlm.nih.gov | |
Description | Data deposited in or computed by PubChem | |
Physical Description |
Liquid, Colorless to pale yellow liquid; [Alfa Aesar MSDS] | |
Record name | 1,2-Ethanediamine, N1-[2-(dimethylamino)ethyl]-N1,N2,N2-trimethyl- | |
Source | EPA Chemicals under the TSCA | |
URL | https://www.epa.gov/chemicals-under-tsca | |
Description | EPA Chemicals under the Toxic Substances Control Act (TSCA) collection contains information on chemicals and their regulations under TSCA, including non-confidential content from the TSCA Chemical Substance Inventory and Chemical Data Reporting. | |
Record name | N,N,N',N',N"-Pentamethyldiethylenetriamine | |
Source | Haz-Map, Information on Hazardous Chemicals and Occupational Diseases | |
URL | https://haz-map.com/Agents/21205 | |
Description | Haz-Map® is an occupational health database designed for health and safety professionals and for consumers seeking information about the adverse effects of workplace exposures to chemical and biological agents. | |
Explanation | Copyright (c) 2022 Haz-Map(R). All rights reserved. Unless otherwise indicated, all materials from Haz-Map are copyrighted by Haz-Map(R). No part of these materials, either text or image may be used for any purpose other than for personal use. Therefore, reproduction, modification, storage in a retrieval system or retransmission, in any form or by any means, electronic, mechanical or otherwise, for reasons other than personal use, is strictly prohibited without prior written permission. | |
Vapor Pressure |
0.3 [mmHg] | |
Record name | N,N,N',N',N"-Pentamethyldiethylenetriamine | |
Source | Haz-Map, Information on Hazardous Chemicals and Occupational Diseases | |
URL | https://haz-map.com/Agents/21205 | |
Description | Haz-Map® is an occupational health database designed for health and safety professionals and for consumers seeking information about the adverse effects of workplace exposures to chemical and biological agents. | |
Explanation | Copyright (c) 2022 Haz-Map(R). All rights reserved. Unless otherwise indicated, all materials from Haz-Map are copyrighted by Haz-Map(R). No part of these materials, either text or image may be used for any purpose other than for personal use. Therefore, reproduction, modification, storage in a retrieval system or retransmission, in any form or by any means, electronic, mechanical or otherwise, for reasons other than personal use, is strictly prohibited without prior written permission. | |
CAS No. |
3030-47-5 | |
Record name | Pentamethyldiethylenetriamine | |
Source | CAS Common Chemistry | |
URL | https://commonchemistry.cas.org/detail?cas_rn=3030-47-5 | |
Description | CAS Common Chemistry is an open community resource for accessing chemical information. Nearly 500,000 chemical substances from CAS REGISTRY cover areas of community interest, including common and frequently regulated chemicals, and those relevant to high school and undergraduate chemistry classes. This chemical information, curated by our expert scientists, is provided in alignment with our mission as a division of the American Chemical Society. | |
Explanation | The data from CAS Common Chemistry is provided under a CC-BY-NC 4.0 license, unless otherwise stated. | |
Record name | 1,2-Ethanediamine, N1-(2-(dimethylamino)ethyl)-N1,N2,N2-trimethyl- | |
Source | ChemIDplus | |
URL | https://pubchem.ncbi.nlm.nih.gov/substance/?source=chemidplus&sourceid=0003030475 | |
Description | ChemIDplus is a free, web search system that provides access to the structure and nomenclature authority files used for the identification of chemical substances cited in National Library of Medicine (NLM) databases, including the TOXNET system. | |
Record name | Pentamethyldiethylenetriamine | |
Source | DTP/NCI | |
URL | https://dtp.cancer.gov/dtpstandard/servlet/dwindex?searchtype=NSC&outputformat=html&searchlist=65659 | |
Description | The NCI Development Therapeutics Program (DTP) provides services and resources to the academic and private-sector research communities worldwide to facilitate the discovery and development of new cancer therapeutic agents. | |
Explanation | Unless otherwise indicated, all text within NCI products is free of copyright and may be reused without our permission. Credit the National Cancer Institute as the source. | |
Record name | 1,2-Ethanediamine, N1-[2-(dimethylamino)ethyl]-N1,N2,N2-trimethyl- | |
Source | EPA Chemicals under the TSCA | |
URL | https://www.epa.gov/chemicals-under-tsca | |
Description | EPA Chemicals under the Toxic Substances Control Act (TSCA) collection contains information on chemicals and their regulations under TSCA, including non-confidential content from the TSCA Chemical Substance Inventory and Chemical Data Reporting. | |
Record name | 1,1,4,7,7-Pentamethyldiethylenetriamine | |
Source | EPA DSSTox | |
URL | https://comptox.epa.gov/dashboard/DTXSID7029249 | |
Description | DSSTox provides a high quality public chemistry resource for supporting improved predictive toxicology. | |
Record name | Bis(2-dimethylaminoethyl)(methyl)amine | |
Source | European Chemicals Agency (ECHA) | |
URL | https://echa.europa.eu/substance-information/-/substanceinfo/100.019.275 | |
Description | The European Chemicals Agency (ECHA) is an agency of the European Union which is the driving force among regulatory authorities in implementing the EU's groundbreaking chemicals legislation for the benefit of human health and the environment as well as for innovation and competitiveness. | |
Explanation | Use of the information, documents and data from the ECHA website is subject to the terms and conditions of this Legal Notice, and subject to other binding limitations provided for under applicable law, the information, documents and data made available on the ECHA website may be reproduced, distributed and/or used, totally or in part, for non-commercial purposes provided that ECHA is acknowledged as the source: "Source: European Chemicals Agency, http://echa.europa.eu/". Such acknowledgement must be included in each copy of the material. ECHA permits and encourages organisations and individuals to create links to the ECHA website under the following cumulative conditions: Links can only be made to webpages that provide a link to the Legal Notice page. | |
Record name | PENTAMETHYLDIETHYLENETRIAMINE | |
Source | FDA Global Substance Registration System (GSRS) | |
URL | https://gsrs.ncats.nih.gov/ginas/app/beta/substances/3274UTY3HL | |
Description | The FDA Global Substance Registration System (GSRS) enables the efficient and accurate exchange of information on what substances are in regulated products. Instead of relying on names, which vary across regulatory domains, countries, and regions, the GSRS knowledge base makes it possible for substances to be defined by standardized, scientific descriptions. | |
Explanation | Unless otherwise noted, the contents of the FDA website (www.fda.gov), both text and graphics, are not copyrighted. They are in the public domain and may be republished, reprinted and otherwise used freely by anyone without the need to obtain permission from FDA. Credit to the U.S. Food and Drug Administration as the source is appreciated but not required. | |
Synthesis routes and methods
Procedure details
Retrosynthesis Analysis
AI-Powered Synthesis Planning: Our tool employs the Template_relevance Pistachio, Template_relevance Bkms_metabolic, Template_relevance Pistachio_ringbreaker, Template_relevance Reaxys, Template_relevance Reaxys_biocatalysis model, leveraging a vast database of chemical reactions to predict feasible synthetic routes.
One-Step Synthesis Focus: Specifically designed for one-step synthesis, it provides concise and direct routes for your target compounds, streamlining the synthesis process.
Accurate Predictions: Utilizing the extensive PISTACHIO, BKMS_METABOLIC, PISTACHIO_RINGBREAKER, REAXYS, REAXYS_BIOCATALYSIS database, our tool offers high-accuracy predictions, reflecting the latest in chemical research and data.
Strategy Settings
Precursor scoring | Relevance Heuristic |
---|---|
Min. plausibility | 0.01 |
Model | Template_relevance |
Template Set | Pistachio/Bkms_metabolic/Pistachio_ringbreaker/Reaxys/Reaxys_biocatalysis |
Top-N result to add to graph | 6 |
Feasible Synthetic Routes
Disclaimer and Information on In-Vitro Research Products
Please be aware that all articles and product information presented on BenchChem are intended solely for informational purposes. The products available for purchase on BenchChem are specifically designed for in-vitro studies, which are conducted outside of living organisms. In-vitro studies, derived from the Latin term "in glass," involve experiments performed in controlled laboratory settings using cells or tissues. It is important to note that these products are not categorized as medicines or drugs, and they have not received approval from the FDA for the prevention, treatment, or cure of any medical condition, ailment, or disease. We must emphasize that any form of bodily introduction of these products into humans or animals is strictly prohibited by law. It is essential to adhere to these guidelines to ensure compliance with legal and ethical standards in research and experimentation.