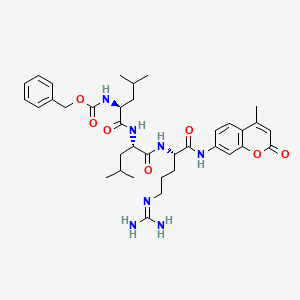
Z-Leu-leu-arg-amc
Overview
Description
Z-Leu-Leu-Arg-AMC (CAS: 90468-17-0) is a fluorogenic tripeptide substrate widely used in enzymatic assays, particularly for studying protease activity. It consists of a Cbz (carbobenzoxy) protecting group at the N-terminus, followed by two leucine residues, an arginine residue, and a C-terminal 7-amino-4-methylcoumarin (AMC) fluorophore. Upon cleavage by proteases such as cathepsin S or Plasmodium falciparum enzymes, the AMC group is released, emitting fluorescence at 460 nm (excitation: 380 nm), enabling real-time monitoring of enzymatic activity .
Mechanism of Action
Target of Action
Z-Leu-leu-arg-amc is a 4-methylcoumarinyl-7-amide (Amc) leucine derivative with carboxybenzoyl (Z). It is the active site of cysteine proteinase trypanopain-Tb from Trypanosoma brucei brucei . It is also the preferred substrate of falcipain II, a hemoglobin-degrading cysteine protease from Plasmodium falciparum , and is used for assaying cathepsin K, an enzyme involved in osteoclastic bone resorption .
Mode of Action
this compound interacts with its targets by serving as a substrate. It is cleaved by the enzymes it targets, such as the cysteine proteinase trypanopain-Tb and falcipain II . This interaction leads to changes in the activity of these enzymes, influencing the processes they are involved in.
Biochemical Pathways
The cleavage of this compound by its target enzymes plays a role in several biochemical pathways. For instance, the cleavage by falcipain II is part of the hemoglobin degradation process in Plasmodium falciparum, which is crucial for the survival and virulence of this malaria parasite . Similarly, the cleavage by cathepsin K is involved in the process of osteoclastic bone resorption .
Result of Action
The cleavage of this compound by its target enzymes results in changes at the molecular and cellular levels. For instance, its cleavage by falcipain II contributes to the degradation of hemoglobin, a process that is essential for the survival of Plasmodium falciparum . Similarly, its cleavage by cathepsin K plays a role in osteoclastic bone resorption, a process that is crucial for bone remodeling .
Biochemical Analysis
Biochemical Properties
Z-Leu-leu-arg-amc plays a crucial role in biochemical reactions as a substrate for various proteases. It is specifically recognized and cleaved by cysteine proteases such as cathepsin B, cathepsin L, and trypanopain-Tb from Trypanosoma brucei brucei . The cleavage of this compound by these enzymes releases the fluorescent AMC moiety, which can be quantitatively measured to assess enzyme activity. This interaction is essential for studying the kinetics and inhibition of proteases, providing insights into their biological functions and potential therapeutic targets.
Cellular Effects
This compound influences various cellular processes by serving as a substrate for proteases involved in protein degradation and turnover. In cellular assays, the cleavage of this compound by cathepsins and other proteases can be used to monitor proteolytic activity within cells . This compound’s impact on cell signaling pathways, gene expression, and cellular metabolism is primarily indirect, as it serves as a tool to study the activity of proteases that regulate these processes.
Molecular Mechanism
The molecular mechanism of this compound involves its recognition and cleavage by specific proteases. The compound binds to the active site of cysteine proteases, where the peptide bond between leucine and arginine is hydrolyzed, releasing the fluorescent AMC moiety . This reaction is highly specific and can be used to measure the activity of proteases in various biological samples. The fluorescence intensity correlates with the amount of substrate cleaved, allowing for quantitative analysis of enzyme activity.
Temporal Effects in Laboratory Settings
In laboratory settings, the stability and degradation of this compound are critical factors influencing its effectiveness as a substrate. The compound is generally stable when stored at -20°C or -80°C, away from moisture and light . Over time, repeated freeze-thaw cycles can lead to degradation, reducing its efficacy. Long-term studies have shown that this compound maintains its activity for several months under proper storage conditions, making it a reliable tool for extended research projects.
Dosage Effects in Animal Models
The effects of this compound in animal models vary with different dosages. In studies involving protease activity assays, the compound is administered at varying concentrations to determine the optimal dosage for accurate measurements . High doses of this compound can lead to saturation of the enzyme’s active site, resulting in non-linear kinetics and potential toxic effects. Therefore, careful titration is necessary to achieve reliable results without adverse effects.
Metabolic Pathways
This compound is primarily involved in metabolic pathways related to protein degradation. It interacts with cysteine proteases, which play a vital role in the breakdown of proteins within cells . The cleavage of this compound by these enzymes releases the AMC moiety, which can be further metabolized or excreted. This process is essential for maintaining cellular homeostasis and regulating protein turnover.
Transport and Distribution
Within cells and tissues, this compound is transported and distributed based on its interactions with proteases and other binding proteins. The compound’s localization is influenced by the presence of specific proteases that recognize and cleave it . This targeted distribution ensures that this compound is primarily active in regions where protease activity is high, allowing for precise measurements of enzyme function.
Subcellular Localization
The subcellular localization of this compound is determined by its interactions with proteases and other cellular components. The compound is typically found in lysosomes and other organelles where protease activity is concentrated . This localization is crucial for its function as a substrate, enabling accurate assessment of protease activity within specific cellular compartments.
Biological Activity
Z-Leu-Leu-Arg-AMC (also known as Z-LLR-AMC) is a synthetic peptide substrate that has garnered significant attention in biochemical research due to its role in studying protease activity. This compound is particularly relevant in cancer research, drug development, and diagnostics. Below, we explore its biological activity, applications, and relevant research findings.
- Molecular Formula : C₃₆H₄₉N₇O₇
- Molecular Weight : 691.82 g/mol
- CAS Number : 90468-17-0
- Structure : this compound is a derivative of 4-methylcoumarinyl-7-amide, which serves as a fluorogenic substrate for various proteases, particularly cathepsins.
Biological Activity
This compound is primarily utilized as a substrate in protease activity assays. Its biological activity can be summarized as follows:
- Protease Substrate : It is specifically designed for measuring the activity of cysteine proteases, such as cathepsin S. This is crucial for understanding metabolic pathways and disease mechanisms, particularly in cancer .
- Drug Development : The compound aids in screening potential drug candidates by providing insights into enzyme interactions. This application is vital for pharmaceutical companies aiming to develop effective therapeutics targeting proteolytic enzymes .
- Biochemical Research : It facilitates studies on protein synthesis and degradation, allowing researchers to explore cellular mechanisms and the effects of various compounds on protein function .
- Diagnostics : this compound can be employed in diagnostic assays to detect diseases associated with specific protease activities, making it a valuable tool for clinical laboratories .
- Biotechnology Applications : In biotechnology, it is used in developing biosensors that detect protease activity, which can be applied across various industrial processes .
Research Findings
Several studies have highlighted the utility and effectiveness of this compound in different contexts:
Case Study 1: Protease Activity Measurement
A study investigated the use of this compound as a substrate for measuring cathepsin S activity in cancer cell lines. The results demonstrated that this substrate allowed for precise quantification of enzyme activity, which correlated with the progression of cancerous conditions .
Case Study 2: Drug Interaction Studies
Another research focused on the interaction of this compound with novel inhibitors of cysteine proteases. The findings indicated that these inhibitors could effectively reduce the cleavage of this compound by cathepsins, suggesting potential therapeutic applications in diseases where these enzymes are upregulated .
Applications Summary Table
Application | Description |
---|---|
Protease Activity Assays | Used to measure enzyme activity in biological samples, crucial for metabolic process studies. |
Drug Development | Assists in screening drug candidates by analyzing enzyme interactions. |
Biochemical Research | Aids in exploring protein synthesis/degradation mechanisms and effects of compounds on proteins. |
Diagnostics | Employed in assays to detect diseases related to specific proteases. |
Biotechnology | Utilized in biosensor development for industrial applications detecting protease activity. |
Scientific Research Applications
Protease Activity Assays
Z-Leu-Leu-Arg-AMC serves as a substrate for various proteases, enabling researchers to measure enzyme activity in biological samples. This application is crucial for understanding metabolic processes and the role of proteases in disease progression.
Drug Development
The compound plays a significant role in the screening of potential drug candidates by providing insights into enzyme interactions. This helps pharmaceutical companies design more effective therapeutics targeting specific proteases.
Biochemical Research
In studies related to protein synthesis and degradation, this compound aids scientists in exploring cellular mechanisms and the effects of different compounds on protein function.
Diagnostics
This compound can be employed in diagnostic assays to detect specific protease-related diseases, offering valuable tools for clinical laboratories. Its ability to indicate protease activity can help diagnose conditions like cancer or infectious diseases.
Biotechnology Applications
In biotechnology, this compound is utilized in developing biosensors that detect protease activity, applicable in various industrial processes, including food safety and pharmaceuticals.
Data Tables
Application | Description | Target Proteases |
---|---|---|
Protease Activity Assays | Measures enzyme activity using fluorescence from AMC upon cleavage | Cathepsins, Kallikreins |
Drug Development | Screens potential drug candidates by analyzing enzyme interactions | Various therapeutic targets |
Biochemical Research | Studies protein synthesis and degradation mechanisms | Cathepsins, Proteasomes |
Diagnostics | Detects protease-related diseases through enzyme activity measurement | Disease-specific proteases |
Biotechnology Applications | Develops biosensors for industrial applications | Various industrial enzymes |
Protease Activity in Cancer Research
This compound has been employed to study the role of cathepsins in cancer progression. Elevated levels of cathepsins have been associated with tumor invasion and metastasis, making this compound a valuable tool for investigating these processes. For instance, a study demonstrated that cathepsin B activity correlates with tumor aggressiveness, highlighting the potential of ZLLR-AMC in cancer diagnostics and therapeutics .
Malaria Research
The interaction of this compound with falcipains (proteases from Plasmodium falciparum) underscores its relevance in malaria research. Understanding these proteases' functions can aid in developing new antimalarial therapies. A study utilizing ZLLR-AMC showed its effectiveness in measuring falcipain activity, which is crucial for identifying potential inhibitors .
Q & A
Basic Research Questions
Q. What experimental protocols are recommended for using Z-Leu-Leu-Arg-AMC in protease activity assays?
this compound is commonly used as a fluorogenic substrate for trypsin-like proteases. Standard protocols involve preparing a reaction buffer (e.g., 50 mM Tris-HCl, pH 8.0, 150 mM NaCl) and optimizing substrate concentration (typically 10–100 µM). Fluorescence intensity (excitation/emission: 380/460 nm) should be monitored over time, with controls for background fluorescence and enzyme autolysis. Ensure calibration with known enzyme activity standards to validate results .
Q. How does this compound compare to other AMC-conjugated substrates in specificity studies?
Specificity depends on the protease’s active site. For example, this compound is selective for calpain-1 over caspase-3 due to its tripeptide sequence. Comparative studies should include parallel assays with substrates like Z-Gly-Pro-AMC (for dipeptidyl peptidases) or Z-Arg-Arg-AMC (for cathepsin B). Use kinetic parameters (Km, kcat) to quantify selectivity differences .
Q. What are the key storage conditions to maintain this compound stability?
Store lyophilized powder at –20°C in a desiccator. Reconstitute in DMSO or DMF (10 mM stock) to prevent hydrolysis. Avoid freeze-thaw cycles; aliquot working solutions and use within 24 hours. Regularly verify purity via HPLC (≥95%) to detect degradation .
Advanced Research Questions
Q. How can researchers resolve discrepancies in kinetic data when using this compound across different assay formats (e.g., plate readers vs. fluorometers)?
Discrepancies often arise from instrument sensitivity variations or temperature inconsistencies. Calibrate instruments with standardized AMC solutions. Perform parallel assays under identical conditions (e.g., path length, gain settings) and normalize data using internal controls (e.g., a reference protease). Statistical tools like Bland-Altman plots can identify systematic biases .
Q. What strategies are effective for integrating this compound into multiplex assays with competing substrates?
Use wavelength-selective fluorophores (e.g., AMC at 460 nm and AFC at 505 nm) to distinguish signals. Optimize substrate concentrations to avoid enzyme saturation and cross-talk. Validate via dose-response curves and include quench controls (e.g., EDTA for metalloproteases) to isolate specific activities .
Q. How should researchers address batch-to-batch variability in this compound purity and its impact on enzyme kinetics?
Source the compound from suppliers with rigorous QC documentation (e.g., HPLC traces, mass spectrometry data). Pre-screen each batch by measuring baseline fluorescence and Km values against a reference enzyme (e.g., trypsin). Adjust substrate concentrations empirically if variability exceeds 10% .
Q. What computational methods support the design of derivatives of this compound for enhanced protease specificity?
Molecular docking (e.g., AutoDock Vina) can model substrate-enzyme interactions to predict binding affinities. Focus on modifying the P1–P3 residues (e.g., replacing Leu with hydrophobic analogs) to alter specificity. Validate predictions with kinetic assays and structural studies (e.g., X-ray crystallography) .
Q. Data Analysis & Contradiction Management
Q. How should researchers interpret conflicting results when this compound activity data contradicts Western blot or genetic knockdown findings?
Cross-validate using orthogonal methods (e.g., activity-based probes or FRET substrates). Consider post-translational modifications or endogenous inhibitors affecting enzyme activity. Use statistical reconciliation (e.g., Bayesian meta-analysis) to weigh evidence from multiple methodologies .
Q. What statistical frameworks are recommended for analyzing time-course fluorescence data from this compound assays?
Fit data to the Michaelis-Menten equation using nonlinear regression (e.g., GraphPad Prism). For complex kinetics (e.g., allosteric regulation), employ global fitting or compartmental models. Report confidence intervals and use Akaike’s Information Criterion (AIC) to compare model fits .
Q. Experimental Design & Optimization
Q. How can researchers minimize photobleaching artifacts in long-term this compound fluorescence assays?
Use low-intensity excitation light and integrate oxygen-scavenging systems (e.g., glucose oxidase/catalase). Include control wells without enzyme to quantify photobleaching rates. For plate readers, employ bottom-read modes to reduce path-length variability .
Q. What are the ethical considerations when designing animal studies using this compound to profile protease activity in disease models?
Follow ARRIVE guidelines for experimental rigor and transparency. Justify sample sizes via power analysis and minimize animal use via longitudinal sampling (e.g., serial blood draws). Include sham controls to distinguish disease-specific protease activity .
Comparison with Similar Compounds
Key Properties:
Property | Value |
---|---|
Molecular Formula | C₃₆H₄₉N₇O₇ |
Molecular Weight | 691.82 g/mol |
Purity | ≥95% (HPLC) |
Storage Conditions | -80°C to -20°C, desiccated |
Applications | Cathepsin S assays, malaria research |
This compound is critical in drug discovery for evaluating protease inhibitors and understanding parasitic enzyme mechanisms .
Comparison with Structurally Similar Compounds
Z-Leu-Leu-Arg-AMC belongs to a class of AMC-conjugated peptide substrates. Below is a comparative analysis with functionally or structurally analogous compounds:
Table 1: Structural and Functional Comparison
Table 2: Enzymatic Activity and Selectivity
Critical Analysis of Differences
- Structural Impact : Substitutions at the P1 position (e.g., Arg → Glu) drastically alter enzyme selectivity. Arg-containing substrates target trypsin-like proteases, while Glu variants bind to aspartic proteases .
- Solubility and Stability : Acetate or HCl salt forms (e.g., Z-Leu-Arg-Gly-Gly-AMC acetate) enhance aqueous solubility, critical for high-throughput screening .
- Assay Compatibility : this compound’s fluorescence signal is pH-sensitive, requiring strict buffer control, whereas Z-Phe-Arg-AMC HCl performs reliably in acidic environments .
Preparation Methods
Solid-Phase Peptide Synthesis (SPPS)
The primary method for preparing Z-Leu-Leu-Arg-AMC is Fmoc-based solid-phase peptide synthesis (SPPS) , a standard technique for assembling peptides with high purity and yield. The process typically includes the following steps:
Resin Loading: The synthesis begins with anchoring the C-terminal amino acid (arginine) onto a solid resin support. The resin used is often a Wang or Rink amide resin, depending on the desired peptide C-terminus.
Fmoc Deprotection: The N-terminal Fmoc protecting group is removed using a mild base such as 20% piperidine in dimethylformamide (DMF), exposing the amine group for coupling.
Amino Acid Coupling: Successive amino acids (leucine, leucine) are coupled using activated derivatives such as Fmoc-Leu-OH with coupling reagents like HBTU/HOBt or DIC/Oxyma. Each coupling is followed by washing and deprotection cycles.
Attachment of the AMC Group: The 7-amino-4-methylcoumarin (AMC) moiety is introduced at the C-terminal arginine amide, usually by coupling the AMC group as an amide or via a pre-activated AMC derivative to the peptide-resin complex.
Cleavage and Deprotection: After completing the peptide chain assembly, the peptide is cleaved from the resin and side-chain protecting groups are removed using a cleavage cocktail, commonly trifluoroacetic acid (TFA) with scavengers like water, triisopropylsilane, and ethanedithiol.
Precipitation and Purification: The crude peptide is precipitated in cold diethyl ether, then purified by reverse-phase high-performance liquid chromatography (RP-HPLC) to achieve high purity.
This method allows precise control over the peptide sequence and the attachment of the AMC fluorophore, which is essential for its function as a protease substrate.
Purification and Characterization
Purification: RP-HPLC is the standard purification technique, using C18 columns with gradients of acetonitrile and water containing 0.1% TFA. Purity is typically confirmed to be >95%.
Characterization: The purified compound is characterized by mass spectrometry (MALDI-TOF or ESI-MS) to confirm molecular weight (this compound has a molecular weight around 578.66 g/mol) and by analytical HPLC to verify purity.
Preparation of Stock Solutions and Formulations
For biochemical assays and in vivo experiments, this compound is prepared as stock solutions and formulated to ensure solubility and stability:
Mass of this compound | Volume of Solvent for 1 mM Solution (mL) | Volume for 5 mM Solution (mL) | Volume for 10 mM Solution (mL) |
---|---|---|---|
1 mg | 1.4454 | 0.2891 | 0.1445 |
5 mg | 7.2272 | 1.4454 | 0.7227 |
10 mg | 14.4544 | 2.8909 | 1.4454 |
Table 1: Stock solution preparation volumes for this compound in DMSO or suitable solvents.
Solvents: Dimethyl sulfoxide (DMSO) is commonly used to prepare master stock solutions due to its high solubilizing power.
In Vivo Formulation: For animal studies, the stock solution is diluted with co-solvents such as polyethylene glycol 300 (PEG300), Tween 80, and water or corn oil to achieve a clear, injectable formulation. The solvents must be added sequentially, ensuring clarity at each step, often aided by vortexing, ultrasound, or mild heating.
Analytical and Quality Control Considerations
Storage: The compound is typically stored at low temperatures (e.g., -20°C) and shipped with cooling agents (blue ice) to maintain stability.
Stability: Stability studies indicate that this compound remains stable in DMSO and formulated solutions when stored properly, but repeated freeze-thaw cycles should be avoided.
Batch Consistency: Each batch is tested for purity, identity, and biological activity to ensure reproducibility in enzymatic assays.
Research Findings on Preparation Optimization
The use of Fmoc-SPPS allows for efficient synthesis with minimal racemization and side reactions.
Incorporation of AMC at the C-terminal arginine is optimized to maintain fluorescence properties without compromising substrate recognition by proteases.
Formulation protocols emphasize the importance of solvent order and clarity to prevent precipitation and ensure bioavailability in vivo.
The compound’s high purity and well-characterized preparation methods support its broad application in protease activity assays, drug screening, and diagnostic research.
Summary Table: Preparation Workflow of this compound
Step | Description | Key Reagents/Conditions | Notes |
---|---|---|---|
1. Resin Loading | Attach Arg to resin | Wang or Rink amide resin | Start of peptide chain assembly |
2. Fmoc Deprotection | Remove Fmoc group | 20% Piperidine in DMF | Expose amine for next coupling |
3. Amino Acid Coupling | Sequential addition of Leu, Leu | Fmoc-Leu-OH, HBTU/HOBt or DIC/Oxyma | Repeat cycles for peptide elongation |
4. AMC Coupling | Attach AMC fluorophore to C-terminal Arg | Pre-activated AMC derivative | Critical for fluorogenic activity |
5. Cleavage & Deprotection | Release peptide and remove side-chain groups | TFA cocktail (TFA, water, scavengers) | Obtain crude peptide |
6. Purification | RP-HPLC purification | C18 column, acetonitrile/water gradient | Achieve >95% purity |
7. Characterization | Mass spectrometry and HPLC | MALDI-TOF or ESI-MS | Confirm identity and purity |
8. Stock Solution Prep | Dissolve in DMSO and dilute for assays/in vivo | DMSO, PEG300, Tween 80, water or corn oil | Ensure solubility and clarity |
9. Storage | Store at low temperature | -20°C, shipped with cooling | Maintain stability |
Properties
IUPAC Name |
benzyl N-[(2S)-1-[[(2S)-1-[[(2S)-5-(diaminomethylideneamino)-1-[(4-methyl-2-oxochromen-7-yl)amino]-1-oxopentan-2-yl]amino]-4-methyl-1-oxopentan-2-yl]amino]-4-methyl-1-oxopentan-2-yl]carbamate | |
---|---|---|
Source | PubChem | |
URL | https://pubchem.ncbi.nlm.nih.gov | |
Description | Data deposited in or computed by PubChem | |
InChI |
InChI=1S/C36H49N7O7/c1-21(2)16-28(42-34(47)29(17-22(3)4)43-36(48)49-20-24-10-7-6-8-11-24)33(46)41-27(12-9-15-39-35(37)38)32(45)40-25-13-14-26-23(5)18-31(44)50-30(26)19-25/h6-8,10-11,13-14,18-19,21-22,27-29H,9,12,15-17,20H2,1-5H3,(H,40,45)(H,41,46)(H,42,47)(H,43,48)(H4,37,38,39)/t27-,28-,29-/m0/s1 | |
Source | PubChem | |
URL | https://pubchem.ncbi.nlm.nih.gov | |
Description | Data deposited in or computed by PubChem | |
InChI Key |
BSZFZMKZCRGANH-AWCRTANDSA-N | |
Source | PubChem | |
URL | https://pubchem.ncbi.nlm.nih.gov | |
Description | Data deposited in or computed by PubChem | |
Canonical SMILES |
CC1=CC(=O)OC2=C1C=CC(=C2)NC(=O)C(CCCN=C(N)N)NC(=O)C(CC(C)C)NC(=O)C(CC(C)C)NC(=O)OCC3=CC=CC=C3 | |
Source | PubChem | |
URL | https://pubchem.ncbi.nlm.nih.gov | |
Description | Data deposited in or computed by PubChem | |
Isomeric SMILES |
CC1=CC(=O)OC2=C1C=CC(=C2)NC(=O)[C@H](CCCN=C(N)N)NC(=O)[C@H](CC(C)C)NC(=O)[C@H](CC(C)C)NC(=O)OCC3=CC=CC=C3 | |
Source | PubChem | |
URL | https://pubchem.ncbi.nlm.nih.gov | |
Description | Data deposited in or computed by PubChem | |
Molecular Formula |
C36H49N7O7 | |
Source | PubChem | |
URL | https://pubchem.ncbi.nlm.nih.gov | |
Description | Data deposited in or computed by PubChem | |
Molecular Weight |
691.8 g/mol | |
Source | PubChem | |
URL | https://pubchem.ncbi.nlm.nih.gov | |
Description | Data deposited in or computed by PubChem | |
Retrosynthesis Analysis
AI-Powered Synthesis Planning: Our tool employs the Template_relevance Pistachio, Template_relevance Bkms_metabolic, Template_relevance Pistachio_ringbreaker, Template_relevance Reaxys, Template_relevance Reaxys_biocatalysis model, leveraging a vast database of chemical reactions to predict feasible synthetic routes.
One-Step Synthesis Focus: Specifically designed for one-step synthesis, it provides concise and direct routes for your target compounds, streamlining the synthesis process.
Accurate Predictions: Utilizing the extensive PISTACHIO, BKMS_METABOLIC, PISTACHIO_RINGBREAKER, REAXYS, REAXYS_BIOCATALYSIS database, our tool offers high-accuracy predictions, reflecting the latest in chemical research and data.
Strategy Settings
Precursor scoring | Relevance Heuristic |
---|---|
Min. plausibility | 0.01 |
Model | Template_relevance |
Template Set | Pistachio/Bkms_metabolic/Pistachio_ringbreaker/Reaxys/Reaxys_biocatalysis |
Top-N result to add to graph | 6 |
Feasible Synthetic Routes
Disclaimer and Information on In-Vitro Research Products
Please be aware that all articles and product information presented on BenchChem are intended solely for informational purposes. The products available for purchase on BenchChem are specifically designed for in-vitro studies, which are conducted outside of living organisms. In-vitro studies, derived from the Latin term "in glass," involve experiments performed in controlled laboratory settings using cells or tissues. It is important to note that these products are not categorized as medicines or drugs, and they have not received approval from the FDA for the prevention, treatment, or cure of any medical condition, ailment, or disease. We must emphasize that any form of bodily introduction of these products into humans or animals is strictly prohibited by law. It is essential to adhere to these guidelines to ensure compliance with legal and ethical standards in research and experimentation.