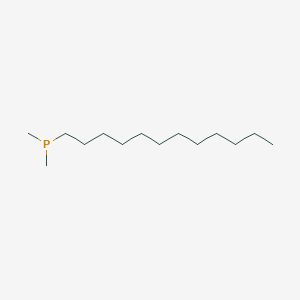
Dodecyl(dimethyl)phosphane
Overview
Description
Dodecyl(dimethyl)phosphane is a tertiary phosphine compound characterized by a central phosphorus atom bonded to a dodecyl (C₁₂H₂₅) chain and two methyl (CH₃) groups. This structure places it within the broader class of organophosphorus compounds, specifically acyclic tertiary phosphanes .
Preparation Methods
Synthetic Routes and Reaction Conditions
Dodecyl(dimethyl)phosphane can be synthesized through several methods. One common approach involves the reaction of dodecyl chloride with dimethylphosphine in the presence of a base such as sodium hydride. The reaction proceeds as follows:
C12H25Cl+(CH3)2PH→C12H25P(CH3)2+HCl
Another method involves the use of Grignard reagents. For example, dodecylmagnesium bromide can react with dimethylphosphine oxide to produce this compound:
C12H25MgBr+(CH3)2P(O)→C12H25P(CH3)2+MgBr(OH)
Industrial Production Methods
Industrial production of this compound typically involves large-scale reactions using similar synthetic routes. The choice of method depends on factors such as cost, availability of reagents, and desired purity of the final product. The reactions are usually carried out in controlled environments to ensure safety and efficiency.
Chemical Reactions Analysis
Types of Reactions
Dodecyl(dimethyl)phosphane undergoes various chemical reactions, including:
Oxidation: It can be oxidized to form dodecyl(dimethyl)phosphine oxide.
Substitution: It can participate in nucleophilic substitution reactions, where the phosphorus atom acts as a nucleophile.
Complexation: It can form complexes with transition metals, which are useful in catalysis.
Common Reagents and Conditions
Oxidation: Common oxidizing agents include hydrogen peroxide and oxygen.
Substitution: Reagents such as alkyl halides and acyl chlorides are used in substitution reactions.
Complexation: Transition metal salts like palladium chloride and platinum chloride are used to form metal-phosphine complexes.
Major Products
Oxidation: Dodecyl(dimethyl)phosphine oxide.
Substitution: Various substituted phosphines depending on the reagents used.
Complexation: Metal-phosphine complexes with potential catalytic applications.
Scientific Research Applications
Dodecyl(dimethyl)phosphane has several applications in scientific research:
Chemistry: It is used as a ligand in coordination chemistry and catalysis. Its ability to form stable complexes with transition metals makes it valuable in catalytic processes.
Biology: It is used in the study of biological membranes and protein interactions. Its amphiphilic nature allows it to interact with lipid bilayers and proteins.
Medicine: Research is ongoing into its potential use in drug delivery systems due to its ability to form stable complexes with various drugs.
Industry: It is used in the production of surfactants and detergents. Its non-ionic nature makes it suitable for use in formulations that require mild detergents.
Mechanism of Action
The mechanism of action of dodecyl(dimethyl)phosphane involves its ability to interact with various molecular targets. In catalysis, it acts as a ligand, coordinating with transition metals to form active catalytic species. In biological systems, it can interact with lipid bilayers and proteins, affecting their structure and function. The specific pathways involved depend on the context in which the compound is used.
Comparison with Similar Compounds
Triphenylphosphane (PPh₃)
- Structure : Tertiary phosphine with three phenyl (C₆H₅) groups attached to phosphorus.
- Applications : Widely used as a ligand in coordination chemistry and catalysis. In gold(I) anticancer compounds, triphenylphosphane ligands contribute to stability and modulate cytotoxicity .
- Reactivity: Acts as a strong σ-donor and weak π-acceptor, facilitating metal-ligand interactions.
- Biological Activity : Gold(I) complexes with triphenylphosphane ligands exhibit IC₅₀ values in the µM range (e.g., 3.46 µM in MDA-MB231 breast cancer cells) and inhibit enzymes like thioredoxin reductase (TrxR) and dihydrofolate reductase (DHFR) .
- Stability : Prone to ligand exchange with cysteine residues in serum albumin, leading to systemic toxicity .
Tridodecyl Phosphite (P(O)(OC₁₂H₂₅)₃)
- Structure : Phosphite ester with three dodecyl groups bonded to phosphorus via oxygen.
- Applications : Used as an antioxidant and stabilizer in polymers.
- Reactivity : Less nucleophilic than phosphines due to electron-withdrawing oxygen atoms.
- Safety: Classified as non-hazardous under normal handling conditions .
Key Difference : Unlike phosphanes, phosphites participate in redox reactions rather than coordination chemistry. Dodecyl(dimethyl)phosphane’s direct P–C bonds make it more reactive toward metal coordination.
Dodecyl Triphenyl Phosphonium Chloride (C₃₀H₄₀ClP)
- Structure : Quaternary phosphonium salt with a dodecyl chain, three phenyl groups, and a chloride counterion.
- Applications : Utilized as a phase-transfer catalyst or ionic liquid due to its charged nature.
- Physicochemical Properties : High molar mass (467.07 g/mol) and ionic character enhance solubility in polar solvents .
Key Difference : Phosphonium salts are ionic and lack the lone pair on phosphorus, rendering them unsuitable as ligands. This compound’s neutral tertiary phosphine structure enables coordination to metal centers.
Gold(I) Phosphane Complexes
- Structure : Gold(I) centers coordinated to phosphane ligands (e.g., [Au(PPh₃)(imidazolate)]).
- Biological Activity : Exhibit cytotoxicity via enzyme inhibition (e.g., TrxR residual activity: 37–49% in SKBR3 cells) .
- Stability Challenges : Susceptible to ligand displacement by serum albumin, necessitating encapsulation strategies to prevent toxicity .
Key Insight : this compound’s hydrophobicity could mitigate albumin interactions, enhancing stability in biological systems compared to hydrophilic analogs.
Data Tables
Table 1: Structural and Functional Comparison of Phosphorus-Containing Compounds
Research Findings and Implications
- Enzyme Inhibition : Phosphane ligands in gold(I) complexes show time-dependent DHFR inhibition, suggesting a dynamic interaction with cellular targets .
- Protein Binding : Strong binding to serum albumin (Ksv = 1.51–2.46 × 10⁴ M⁻¹) highlights the need for encapsulation to improve pharmacokinetics .
- Structural Tuning : The hydrophobicity of this compound could reduce unintended protein interactions compared to triphenylphosphane, making it a candidate for targeted drug delivery.
Q & A
Basic Research Questions
Q. What are the common synthetic routes for preparing Dodecyl(dimethyl)phosphane, and how can reaction conditions be optimized?
- Methodological Answer : The synthesis typically involves nucleophilic substitution using organolithium reagents (e.g., n-BuLi) with chlorodimethylphosphane in anhydrous solvents like diethyl ether or THF at low temperatures (−78°C). For example, details a similar synthesis where chlorodimethylphosphane reacts with aryl lithium intermediates to form phosphane derivatives. Optimization includes controlling stoichiometry, ensuring inert atmospheres, and slow warming to room temperature to avoid side reactions. Post-synthesis purification via distillation or recrystallization is critical, and yields can exceed 85% under controlled conditions .
Q. How should researchers characterize the purity and structure of this compound?
- Methodological Answer : Multinuclear NMR spectroscopy (¹H, ³¹P, ¹⁹F if fluorinated analogs exist) is essential for structural confirmation. For instance, reports ³¹P NMR chemical shifts near −48 ppm for dimethyl(pentafluorophenyl)phosphane, with distinct splitting patterns due to P–F coupling. Elemental analysis (C, H, P) and GC-MS can validate purity. Cross-referencing experimental data with computational predictions (e.g., DFT-calculated NMR shifts) enhances reliability .
Q. What computational methods are suitable for predicting the electronic properties of this compound?
- Methodological Answer : Density Functional Theory (DFT) with hybrid functionals (e.g., B3LYP) and triple-zeta basis sets (e.g., def2-TZVP) provides accurate electronic structure data. Basis sets must balance accuracy and computational cost; recommends split-valance or triple-zeta sets for thermochemical properties. Solvent effects can be modeled using continuum solvation models (e.g., SMD). Software like Gaussian or ORCA is commonly used .
Advanced Research Questions
Q. How does the electronic structure of this compound influence its coordination behavior in catalytic systems?
- Methodological Answer : The electron-donating dodecyl and methyl groups increase the phosphane’s electron density, enhancing its ability to stabilize low-valent metal centers (e.g., Rh or Co in hydroformylation). demonstrates that phosphane ligands with tailored electron-donating groups improve catalytic turnover by stabilizing active intermediates. Researchers should compare catalytic rates using Hammett parameters or DFT-calculated Natural Bond Orbital (NBO) charges to quantify electronic effects .
Q. What strategies resolve discrepancies between experimental and computational data for phosphane ligand geometries?
- Methodological Answer : Discrepancies often arise from inadequate basis sets or neglected dispersion forces. suggests incorporating exact exchange (e.g., in the B3LYP functional) and gradient corrections for improved accuracy. For geometric mismatches, refine computational models by including solvent interactions (via COSMO) or using high-level wavefunction methods (e.g., MP2). Experimental validation via X-ray crystallography (as in ) is critical for benchmarking .
Q. How can researchers design phosphane-based catalysts for aqueous-phase reactions involving hydrophobic substrates?
- Methodological Answer : highlights the use of cyclodextrin-phosphane grafted polymers to enhance substrate solubility and catalytic efficiency. The proximity of phosphane ligands and hydrophobic pockets in such systems accelerates reaction kinetics. Researchers should employ techniques like dynamic light scattering (DLS) to confirm supramolecular assembly and monitor reaction progress via in situ IR or GC-MS .
Q. What kinetic and mechanistic studies are essential for understanding P–C bond formation involving this compound?
- Methodological Answer : Rate laws and isotopic labeling (e.g., deuterated substrates) can elucidate mechanisms. identifies scenarios where phosphane ligand exchange or oxidative addition is rate-determining. Stopped-flow NMR or time-resolved spectroscopy can capture transient intermediates. For example, variable-temperature kinetics combined with Eyring analysis provides activation parameters (ΔH‡, ΔS‡) .
Q. Data Analysis and Contradiction Management
Q. How should researchers address inconsistencies in NMR data for phosphane derivatives?
- Methodological Answer : Contradictions may arise from impurities or dynamic processes (e.g., fluxionality). Purify samples via column chromatography or repeated crystallizations. Variable-temperature NMR can identify dynamic behavior, while 2D experiments (e.g., HSQC, NOESY) resolve overlapping signals. Cross-validate with X-ray structures (as in ) or high-resolution MS .
Q. What methods validate the thermochemical accuracy of DFT models for phosphane-containing systems?
- Methodological Answer : Compare computed bond dissociation energies or reaction enthalpies with experimental calorimetry data. emphasizes using benchmark sets (e.g., G2/97) to assess functional performance. For phosphanes, include dispersion-corrected functionals (e.g., B3LYP-D3) to account for van der Waals interactions in bulky ligands .
Properties
IUPAC Name |
dodecyl(dimethyl)phosphane | |
---|---|---|
Details | Computed by LexiChem 2.6.6 (PubChem release 2019.06.18) | |
Source | PubChem | |
URL | https://pubchem.ncbi.nlm.nih.gov | |
Description | Data deposited in or computed by PubChem | |
InChI |
InChI=1S/C14H31P/c1-4-5-6-7-8-9-10-11-12-13-14-15(2)3/h4-14H2,1-3H3 | |
Details | Computed by InChI 1.0.5 (PubChem release 2019.06.18) | |
Source | PubChem | |
URL | https://pubchem.ncbi.nlm.nih.gov | |
Description | Data deposited in or computed by PubChem | |
InChI Key |
RIXFPXRYYYJJKF-UHFFFAOYSA-N | |
Details | Computed by InChI 1.0.5 (PubChem release 2019.06.18) | |
Source | PubChem | |
URL | https://pubchem.ncbi.nlm.nih.gov | |
Description | Data deposited in or computed by PubChem | |
Canonical SMILES |
CCCCCCCCCCCCP(C)C | |
Details | Computed by OEChem 2.1.5 (PubChem release 2019.06.18) | |
Source | PubChem | |
URL | https://pubchem.ncbi.nlm.nih.gov | |
Description | Data deposited in or computed by PubChem | |
Molecular Formula |
C14H31P | |
Details | Computed by PubChem 2.1 (PubChem release 2019.06.18) | |
Source | PubChem | |
URL | https://pubchem.ncbi.nlm.nih.gov | |
Description | Data deposited in or computed by PubChem | |
DSSTOX Substance ID |
DTXSID00633650 | |
Record name | Dodecyl(dimethyl)phosphane | |
Source | EPA DSSTox | |
URL | https://comptox.epa.gov/dashboard/DTXSID00633650 | |
Description | DSSTox provides a high quality public chemistry resource for supporting improved predictive toxicology. | |
Molecular Weight |
230.37 g/mol | |
Details | Computed by PubChem 2.1 (PubChem release 2021.05.07) | |
Source | PubChem | |
URL | https://pubchem.ncbi.nlm.nih.gov | |
Description | Data deposited in or computed by PubChem | |
CAS No. |
6794-87-2 | |
Record name | Dodecyl(dimethyl)phosphane | |
Source | EPA DSSTox | |
URL | https://comptox.epa.gov/dashboard/DTXSID00633650 | |
Description | DSSTox provides a high quality public chemistry resource for supporting improved predictive toxicology. | |
Disclaimer and Information on In-Vitro Research Products
Please be aware that all articles and product information presented on BenchChem are intended solely for informational purposes. The products available for purchase on BenchChem are specifically designed for in-vitro studies, which are conducted outside of living organisms. In-vitro studies, derived from the Latin term "in glass," involve experiments performed in controlled laboratory settings using cells or tissues. It is important to note that these products are not categorized as medicines or drugs, and they have not received approval from the FDA for the prevention, treatment, or cure of any medical condition, ailment, or disease. We must emphasize that any form of bodily introduction of these products into humans or animals is strictly prohibited by law. It is essential to adhere to these guidelines to ensure compliance with legal and ethical standards in research and experimentation.