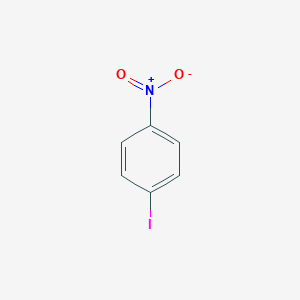
1-Iodo-4-nitrobenzene
Overview
Description
1-Iodo-4-nitrobenzene, also known as p-iodonitrobenzene, is an organic compound with the molecular formula C6H4INO2. It is a yellow crystalline solid with a pungent odor. This compound is widely used as an intermediate in organic synthesis, particularly in the production of dyes, pharmaceuticals, and agrochemicals .
Mechanism of Action
Target of Action
1-Iodo-4-nitrobenzene is a generic chemical reagent used in organic synthesis . The primary targets of this compound are typically organic molecules that it reacts with during these synthesis processes.
Mode of Action
The mode of action of this compound involves its interaction with other molecules in a chemical reaction. For instance, in the hydrogenation of this compound, a sigma-bond is formed with the benzene ring, generating a positively charged benzenonium intermediate . This intermediate then undergoes further reactions.
Biochemical Pathways
The specific biochemical pathways affected by this compound depend on the context of the organic synthesis in which it is used. For example, in the hydrogenation of this compound, it is involved in the reduction of nitro groups to amines .
Result of Action
The result of this compound’s action is typically the formation of a new organic compound. For instance, in the hydrogenation process, the result is the formation of 4-iodoaniline .
Action Environment
The action, efficacy, and stability of this compound can be influenced by various environmental factors. For instance, the temperature of the reaction can significantly affect the rate and outcome of the reaction . Additionally, it is sensitive to light and should be stored in cool, dry conditions in well-sealed containers .
Preparation Methods
Synthetic Routes and Reaction Conditions: 1-Iodo-4-nitrobenzene can be synthesized through the diazotization of 4-nitroaniline followed by iodination. The process involves the following steps:
- Dissolve 0.5 grams of 4-nitroaniline in a mixture of concentrated sulfuric acid and water. Cool the solution to 0-5°C.
- Add a cold solution of sodium nitrite to the mixture to form the diazonium salt.
- Filter the cold solution and add it to a solution of potassium iodide in water with stirring.
- Filter the product, wash it with water, and dry it. The crude product can be recrystallized from ethanol to obtain pure this compound .
Industrial Production Methods: In industrial settings, the synthesis of this compound follows similar principles but is scaled up and optimized for efficiency. The use of continuous flow processes and advanced catalysts, such as Ru-Me/Al2O3, can enhance the yield and purity of the product .
Chemical Reactions Analysis
1-Iodo-4-nitrobenzene undergoes various chemical reactions, including:
Substitution: The iodine atom can be substituted with other groups through reactions with nucleophiles.
Oxidation: The compound can be oxidized under specific conditions, although this is less common.
Common Reagents and Conditions:
- Hydrogenation reactions typically use molecular hydrogen and metal catalysts.
- Substitution reactions often involve nucleophiles like thiols and palladium or nickel catalysts.
Major Products:
- Reduction yields 4-iodoaniline.
- Substitution reactions can produce various aryl derivatives depending on the nucleophile used.
Scientific Research Applications
1-Iodo-4-nitrobenzene is utilized in several scientific research fields:
Biology: The compound is used in the study of enzyme-catalyzed reactions and as a probe for investigating biochemical pathways.
Medicine: It is involved in the development of new drugs and therapeutic agents, particularly those targeting specific enzymes or receptors.
Industry: The compound is used in the production of dyes, pigments, and other industrial chemicals.
Comparison with Similar Compounds
1-Iodo-4-nitrobenzene can be compared with other halogenated nitrobenzenes, such as:
1-Bromo-4-nitrobenzene: Similar in structure but contains a bromine atom instead of iodine. It is less reactive in substitution reactions due to the lower reactivity of the carbon-bromine bond.
1-Chloro-4-nitrobenzene: Contains a chlorine atom and is even less reactive than the bromo and iodo derivatives.
1-Fluoro-4-nitrobenzene: Contains a fluorine atom and is the least reactive among the halogenated nitrobenzenes due to the strong carbon-fluorine bond.
The uniqueness of this compound lies in its higher reactivity, making it a valuable intermediate for various synthetic applications.
Biological Activity
1-Iodo-4-nitrobenzene, a halogenated aromatic compound, has garnered interest in various fields of biological research due to its unique chemical properties and potential applications. This article delves into the biological activity of this compound, exploring its synthesis, mechanisms of action, and relevant case studies.
This compound (CAS No. 636-98-6) is characterized by the presence of iodine and nitro groups on a benzene ring. The synthesis of this compound can involve various methods, including electrophilic aromatic substitution reactions and nucleophilic substitutions. One notable method involves the reaction of arylhydrazines with iodine under metal-free conditions, which offers a straightforward approach to producing aryl iodides like this compound .
Biological Activity Overview
The biological activity of this compound has been studied in several contexts, particularly its cytotoxicity, antimicrobial properties, and effects on cellular mechanisms.
Cytotoxicity
Research indicates that this compound exhibits significant cytotoxic effects on various cancer cell lines. For instance, studies have shown that it can induce apoptosis in breast cancer cells (MCF-7) through the activation of caspase pathways. The compound's IC50 values in different cancer cell lines suggest moderate to high cytotoxicity, making it a candidate for further investigation in cancer therapeutics .
Antimicrobial Activity
The antimicrobial properties of this compound have been evaluated against several bacterial strains. It has demonstrated effectiveness against Gram-positive bacteria such as Staphylococcus aureus and Gram-negative bacteria like Escherichia coli. The minimum inhibitory concentration (MIC) values indicate that the compound can inhibit bacterial growth at relatively low concentrations, suggesting potential use as an antibacterial agent .
The mechanisms through which this compound exerts its biological effects are complex and multifaceted:
- Reactive Oxygen Species (ROS) Generation : The compound is known to induce oxidative stress in cells, leading to increased levels of ROS. This oxidative stress can damage cellular components, including lipids, proteins, and DNA, ultimately resulting in cell death.
- Apoptotic Pathways : Activation of apoptotic pathways has been observed in studies involving cancer cell lines. The compound triggers mitochondrial dysfunction and activates caspases, which are critical mediators of apoptosis.
- Inhibition of Enzymatic Activity : Some studies suggest that this compound may inhibit specific enzymes involved in cellular signaling pathways, although further research is needed to elucidate these interactions fully.
Case Studies
Several key studies highlight the biological activity of this compound:
- Study on Cancer Cell Lines : A study conducted on various cancer cell lines demonstrated that treatment with this compound resulted in significant cell death compared to untreated controls. The study reported IC50 values ranging from 20 to 50 µM across different cell types .
- Antimicrobial Efficacy : In another study assessing the antimicrobial properties, this compound was tested against a panel of bacterial strains. The results indicated that it had an MIC value of 32 µg/mL against Staphylococcus aureus, demonstrating its potential as an antibacterial agent .
Summary Table of Biological Activities
Properties
IUPAC Name |
1-iodo-4-nitrobenzene | |
---|---|---|
Source | PubChem | |
URL | https://pubchem.ncbi.nlm.nih.gov | |
Description | Data deposited in or computed by PubChem | |
InChI |
InChI=1S/C6H4INO2/c7-5-1-3-6(4-2-5)8(9)10/h1-4H | |
Source | PubChem | |
URL | https://pubchem.ncbi.nlm.nih.gov | |
Description | Data deposited in or computed by PubChem | |
InChI Key |
SCCCFNJTCDSLCY-UHFFFAOYSA-N | |
Source | PubChem | |
URL | https://pubchem.ncbi.nlm.nih.gov | |
Description | Data deposited in or computed by PubChem | |
Canonical SMILES |
C1=CC(=CC=C1[N+](=O)[O-])I | |
Source | PubChem | |
URL | https://pubchem.ncbi.nlm.nih.gov | |
Description | Data deposited in or computed by PubChem | |
Molecular Formula |
C6H4INO2 | |
Source | PubChem | |
URL | https://pubchem.ncbi.nlm.nih.gov | |
Description | Data deposited in or computed by PubChem | |
DSSTOX Substance ID |
DTXSID7060914 | |
Record name | Benzene, 1-iodo-4-nitro- | |
Source | EPA DSSTox | |
URL | https://comptox.epa.gov/dashboard/DTXSID7060914 | |
Description | DSSTox provides a high quality public chemistry resource for supporting improved predictive toxicology. | |
Molecular Weight |
249.01 g/mol | |
Source | PubChem | |
URL | https://pubchem.ncbi.nlm.nih.gov | |
Description | Data deposited in or computed by PubChem | |
Physical Description |
Yellow powder; [Alfa Aesar MSDS] | |
Record name | 1-Iodo-4-nitrobenzene | |
Source | Haz-Map, Information on Hazardous Chemicals and Occupational Diseases | |
URL | https://haz-map.com/Agents/19377 | |
Description | Haz-Map® is an occupational health database designed for health and safety professionals and for consumers seeking information about the adverse effects of workplace exposures to chemical and biological agents. | |
Explanation | Copyright (c) 2022 Haz-Map(R). All rights reserved. Unless otherwise indicated, all materials from Haz-Map are copyrighted by Haz-Map(R). No part of these materials, either text or image may be used for any purpose other than for personal use. Therefore, reproduction, modification, storage in a retrieval system or retransmission, in any form or by any means, electronic, mechanical or otherwise, for reasons other than personal use, is strictly prohibited without prior written permission. | |
CAS No. |
636-98-6 | |
Record name | 1-Iodo-4-nitrobenzene | |
Source | CAS Common Chemistry | |
URL | https://commonchemistry.cas.org/detail?cas_rn=636-98-6 | |
Description | CAS Common Chemistry is an open community resource for accessing chemical information. Nearly 500,000 chemical substances from CAS REGISTRY cover areas of community interest, including common and frequently regulated chemicals, and those relevant to high school and undergraduate chemistry classes. This chemical information, curated by our expert scientists, is provided in alignment with our mission as a division of the American Chemical Society. | |
Explanation | The data from CAS Common Chemistry is provided under a CC-BY-NC 4.0 license, unless otherwise stated. | |
Record name | Benzene, 1-iodo-4-nitro- | |
Source | ChemIDplus | |
URL | https://pubchem.ncbi.nlm.nih.gov/substance/?source=chemidplus&sourceid=0000636986 | |
Description | ChemIDplus is a free, web search system that provides access to the structure and nomenclature authority files used for the identification of chemical substances cited in National Library of Medicine (NLM) databases, including the TOXNET system. | |
Record name | 1-Iodo-4-nitrobenzene | |
Source | DTP/NCI | |
URL | https://dtp.cancer.gov/dtpstandard/servlet/dwindex?searchtype=NSC&outputformat=html&searchlist=9794 | |
Description | The NCI Development Therapeutics Program (DTP) provides services and resources to the academic and private-sector research communities worldwide to facilitate the discovery and development of new cancer therapeutic agents. | |
Explanation | Unless otherwise indicated, all text within NCI products is free of copyright and may be reused without our permission. Credit the National Cancer Institute as the source. | |
Record name | Benzene, 1-iodo-4-nitro- | |
Source | EPA Chemicals under the TSCA | |
URL | https://www.epa.gov/chemicals-under-tsca | |
Description | EPA Chemicals under the Toxic Substances Control Act (TSCA) collection contains information on chemicals and their regulations under TSCA, including non-confidential content from the TSCA Chemical Substance Inventory and Chemical Data Reporting. | |
Record name | Benzene, 1-iodo-4-nitro- | |
Source | EPA DSSTox | |
URL | https://comptox.epa.gov/dashboard/DTXSID7060914 | |
Description | DSSTox provides a high quality public chemistry resource for supporting improved predictive toxicology. | |
Record name | 1-iodo-4-nitrobenzene | |
Source | European Chemicals Agency (ECHA) | |
URL | https://echa.europa.eu/substance-information/-/substanceinfo/100.010.248 | |
Description | The European Chemicals Agency (ECHA) is an agency of the European Union which is the driving force among regulatory authorities in implementing the EU's groundbreaking chemicals legislation for the benefit of human health and the environment as well as for innovation and competitiveness. | |
Explanation | Use of the information, documents and data from the ECHA website is subject to the terms and conditions of this Legal Notice, and subject to other binding limitations provided for under applicable law, the information, documents and data made available on the ECHA website may be reproduced, distributed and/or used, totally or in part, for non-commercial purposes provided that ECHA is acknowledged as the source: "Source: European Chemicals Agency, http://echa.europa.eu/". Such acknowledgement must be included in each copy of the material. ECHA permits and encourages organisations and individuals to create links to the ECHA website under the following cumulative conditions: Links can only be made to webpages that provide a link to the Legal Notice page. | |
Record name | 4-Iodonitrobenzene | |
Source | FDA Global Substance Registration System (GSRS) | |
URL | https://gsrs.ncats.nih.gov/ginas/app/beta/substances/NH3N3MPW9D | |
Description | The FDA Global Substance Registration System (GSRS) enables the efficient and accurate exchange of information on what substances are in regulated products. Instead of relying on names, which vary across regulatory domains, countries, and regions, the GSRS knowledge base makes it possible for substances to be defined by standardized, scientific descriptions. | |
Explanation | Unless otherwise noted, the contents of the FDA website (www.fda.gov), both text and graphics, are not copyrighted. They are in the public domain and may be republished, reprinted and otherwise used freely by anyone without the need to obtain permission from FDA. Credit to the U.S. Food and Drug Administration as the source is appreciated but not required. | |
Retrosynthesis Analysis
AI-Powered Synthesis Planning: Our tool employs the Template_relevance Pistachio, Template_relevance Bkms_metabolic, Template_relevance Pistachio_ringbreaker, Template_relevance Reaxys, Template_relevance Reaxys_biocatalysis model, leveraging a vast database of chemical reactions to predict feasible synthetic routes.
One-Step Synthesis Focus: Specifically designed for one-step synthesis, it provides concise and direct routes for your target compounds, streamlining the synthesis process.
Accurate Predictions: Utilizing the extensive PISTACHIO, BKMS_METABOLIC, PISTACHIO_RINGBREAKER, REAXYS, REAXYS_BIOCATALYSIS database, our tool offers high-accuracy predictions, reflecting the latest in chemical research and data.
Strategy Settings
Precursor scoring | Relevance Heuristic |
---|---|
Min. plausibility | 0.01 |
Model | Template_relevance |
Template Set | Pistachio/Bkms_metabolic/Pistachio_ringbreaker/Reaxys/Reaxys_biocatalysis |
Top-N result to add to graph | 6 |
Feasible Synthetic Routes
Disclaimer and Information on In-Vitro Research Products
Please be aware that all articles and product information presented on BenchChem are intended solely for informational purposes. The products available for purchase on BenchChem are specifically designed for in-vitro studies, which are conducted outside of living organisms. In-vitro studies, derived from the Latin term "in glass," involve experiments performed in controlled laboratory settings using cells or tissues. It is important to note that these products are not categorized as medicines or drugs, and they have not received approval from the FDA for the prevention, treatment, or cure of any medical condition, ailment, or disease. We must emphasize that any form of bodily introduction of these products into humans or animals is strictly prohibited by law. It is essential to adhere to these guidelines to ensure compliance with legal and ethical standards in research and experimentation.