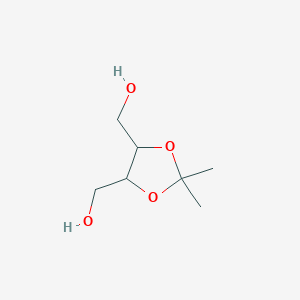
(+)-2,3-O-Isopropylidene-L-threitol
Overview
Description
(+)-2,3-O-Isopropylidene-L-threitol is a chiral organic compound derived from L-threitol. It is characterized by the presence of an isopropylidene group protecting the hydroxyl groups at the 2 and 3 positions. This compound is often used in organic synthesis due to its stereochemical properties and ability to form stable intermediates.
Preparation Methods
Synthetic Routes and Reaction Conditions
(+)-2,3-O-Isopropylidene-L-threitol can be synthesized through the protection of L-threitol using acetone in the presence of an acid catalyst. The reaction typically involves dissolving L-threitol in acetone and adding a catalytic amount of an acid such as sulfuric acid or p-toluenesulfonic acid. The mixture is then stirred at room temperature or slightly elevated temperatures until the reaction is complete. The product is isolated by removing the solvent and purifying the residue through recrystallization or chromatography.
Industrial Production Methods
Industrial production of this compound follows similar principles but on a larger scale. The process involves the use of industrial-grade acetone and acid catalysts in large reactors. The reaction conditions are optimized for maximum yield and purity, and the product is typically purified using industrial-scale chromatography or crystallization techniques.
Chemical Reactions Analysis
Types of Reactions
(+)-2,3-O-Isopropylidene-L-threitol undergoes various chemical reactions, including:
Oxidation: The hydroxyl groups can be oxidized to form ketones or carboxylic acids.
Reduction: The compound can be reduced to form diols or other reduced derivatives.
Substitution: The hydroxyl groups can be substituted with other functional groups using appropriate reagents.
Common Reagents and Conditions
Oxidation: Common oxidizing agents include pyridinium chlorochromate (PCC) and potassium permanganate (KMnO4).
Reduction: Reducing agents such as lithium aluminum hydride (LiAlH4) or sodium borohydride (NaBH4) are commonly used.
Substitution: Reagents like tosyl chloride (TsCl) or mesyl chloride (MsCl) can be used to substitute the hydroxyl groups with tosyl or mesyl groups.
Major Products Formed
Oxidation: Ketones or carboxylic acids.
Reduction: Diols or other reduced derivatives.
Substitution: Tosylates or mesylates.
Scientific Research Applications
Synthetic Organic Chemistry
(+)-2,3-O-Isopropylidene-L-threitol serves as a versatile intermediate in the synthesis of complex organic molecules. Its role is crucial in the development of pharmaceuticals and agrochemicals. The compound facilitates the production of various derivatives that are essential for drug formulation and agricultural products.
Application Area | Description |
---|---|
Pharmaceuticals | Used to synthesize active pharmaceutical ingredients (APIs) through various chemical transformations. |
Agrochemicals | Acts as an intermediate in the synthesis of pesticides and herbicides. |
Chiral Auxiliary in Asymmetric Synthesis
This compound is employed as a chiral auxiliary to enhance the enantioselectivity of reactions, allowing for the efficient production of enantiomerically pure compounds. This is particularly significant in drug development, where the chirality of a molecule can influence its biological activity.
Case Study:
A study demonstrated the use of this compound in synthesizing (-)-anisomycin, highlighting its effectiveness as a chiral precursor .
Bioconjugation Techniques
The compound is utilized in bioconjugation processes, which involve attaching biomolecules to surfaces or other molecules. This application is vital for improving drug delivery systems and developing targeted therapies.
Technique | Description |
---|---|
Bioconjugation | Enhances the targeting and efficacy of therapeutic agents by linking them to specific biomolecules. |
Research in Glycochemistry
This compound plays a role in synthesizing glycosides and other carbohydrate derivatives. These derivatives are essential for studying biological processes and developing carbohydrate-based drugs.
Example Application:
The synthesis of l-threitol-based crown ethers has been explored for their application as phase transfer catalysts in asymmetric Michael addition reactions, demonstrating their utility in catalysis .
Mechanism of Action
The mechanism of action of (+)-2,3-O-Isopropylidene-L-threitol involves its ability to act as a chiral template in chemical reactions. The isopropylidene group protects the hydroxyl groups, allowing for selective reactions at other positions. This protection is crucial in multi-step synthesis processes where selective reactivity is required. The compound’s stereochemistry plays a significant role in determining the outcome of reactions, making it a valuable tool in asymmetric synthesis.
Comparison with Similar Compounds
(+)-2,3-O-Isopropylidene-L-threitol is unique due to its specific stereochemistry and the presence of the isopropylidene protecting group. Similar compounds include:
(+)-2,3-O-Isopropylidene-D-threitol: The enantiomer of this compound.
2,3-O-Isopropylidene-D-glyceraldehyde: A related compound with a similar protecting group but different stereochemistry.
2,3-O-Isopropylidene-L-glyceraldehyde: Another related compound with different stereochemistry.
These compounds share similar chemical properties but differ in their stereochemistry, which affects their reactivity and applications in synthesis.
Biological Activity
(+)-2,3-O-Isopropylidene-L-threitol is a chiral compound that serves as an important intermediate in organic synthesis, particularly in the fields of pharmaceuticals and glycoside formation. Its unique structure allows it to participate in various biochemical reactions, making it a valuable compound for research and application in medicinal chemistry.
- Molecular Formula : C9H18O5
- Molecular Weight : 190.24 g/mol
- Melting Point : 45°C to 49°C
- Boiling Point : 92°C to 94°C (0.1 mmHg)
- Solubility : Soluble in water; hygroscopic nature.
Biological Applications
The biological activity of this compound primarily stems from its role as a precursor in the synthesis of various biologically active compounds. Some notable applications include:
- Glycosylation Reactions : It is frequently used in glycosylation reactions due to its ability to form glycosidic bonds, which are critical in the synthesis of oligosaccharides and glycoconjugates.
- Pharmaceutical Intermediates : The compound is utilized in the development of various pharmaceutical agents, particularly those targeting glycosylation pathways.
While specific mechanisms of action for this compound have not been extensively characterized, its role as a chiral building block suggests that it can influence the stereochemistry of resultant compounds. This stereochemical control is crucial for the biological activity of many drugs.
Research Findings
Recent studies have highlighted several aspects of the biological activity associated with this compound:
- Enzyme Interactions : Research indicates that derivatives of this compound can interact with various enzymes involved in metabolic pathways, potentially affecting their activity and regulation.
- Antiviral Activity : Some derivatives have shown promise in inhibiting viral replication, suggesting that modifications to the isopropylidene structure may enhance antiviral properties.
- Anticancer Potential : Investigations into its derivatives have revealed potential anticancer activities, particularly through mechanisms involving apoptosis and cell cycle regulation.
Case Studies
Several case studies illustrate the compound's application in biological research:
- Study on Glycosylation Efficiency : A study demonstrated that this compound significantly improves the efficiency of glycosylation reactions when used as a donor substrate, resulting in higher yields of desired glycosides.
- Antiviral Compound Development : Researchers synthesized a series of compounds based on this compound and evaluated their antiviral activity against influenza virus. The results indicated that certain modifications enhanced efficacy against viral replication.
Comparative Analysis with Similar Compounds
The following table summarizes key features and applications of related compounds:
Compound Name | Molecular Formula | Key Features | Applications |
---|---|---|---|
This compound | C9H18O5 | Chiral building block for glycosides | Organic synthesis, pharmaceuticals |
(-)-1,4-Di-O-tosyl-2,3-O-isopropylidene-L-threitol | C21H26O8S2 | Enhanced reactivity due to tosyl groups | Glycobiology research |
2,3-O-Isopropylidene-D-threitol | C9H18O5 | Simpler structure without protective groups | Deprotection reactions |
Properties
IUPAC Name |
[(4S,5S)-5-(hydroxymethyl)-2,2-dimethyl-1,3-dioxolan-4-yl]methanol | |
---|---|---|
Source | PubChem | |
URL | https://pubchem.ncbi.nlm.nih.gov | |
Description | Data deposited in or computed by PubChem | |
InChI |
InChI=1S/C7H14O4/c1-7(2)10-5(3-8)6(4-9)11-7/h5-6,8-9H,3-4H2,1-2H3/t5-,6-/m0/s1 | |
Source | PubChem | |
URL | https://pubchem.ncbi.nlm.nih.gov | |
Description | Data deposited in or computed by PubChem | |
InChI Key |
INVRLGIKFANLFP-WDSKDSINSA-N | |
Source | PubChem | |
URL | https://pubchem.ncbi.nlm.nih.gov | |
Description | Data deposited in or computed by PubChem | |
Canonical SMILES |
CC1(OC(C(O1)CO)CO)C | |
Source | PubChem | |
URL | https://pubchem.ncbi.nlm.nih.gov | |
Description | Data deposited in or computed by PubChem | |
Isomeric SMILES |
CC1(O[C@H]([C@@H](O1)CO)CO)C | |
Source | PubChem | |
URL | https://pubchem.ncbi.nlm.nih.gov | |
Description | Data deposited in or computed by PubChem | |
Molecular Formula |
C7H14O4 | |
Source | PubChem | |
URL | https://pubchem.ncbi.nlm.nih.gov | |
Description | Data deposited in or computed by PubChem | |
DSSTOX Substance ID |
DTXSID40964859 | |
Record name | (2,2-Dimethyl-1,3-dioxolane-4,5-diyl)dimethanol | |
Source | EPA DSSTox | |
URL | https://comptox.epa.gov/dashboard/DTXSID40964859 | |
Description | DSSTox provides a high quality public chemistry resource for supporting improved predictive toxicology. | |
Molecular Weight |
162.18 g/mol | |
Source | PubChem | |
URL | https://pubchem.ncbi.nlm.nih.gov | |
Description | Data deposited in or computed by PubChem | |
CAS No. |
86992-57-6, 50622-09-8 | |
Record name | rel-(4R,5R)-2,2-Dimethyl-1,3-dioxolane-4,5-dimethanol | |
Source | CAS Common Chemistry | |
URL | https://commonchemistry.cas.org/detail?cas_rn=86992-57-6 | |
Description | CAS Common Chemistry is an open community resource for accessing chemical information. Nearly 500,000 chemical substances from CAS REGISTRY cover areas of community interest, including common and frequently regulated chemicals, and those relevant to high school and undergraduate chemistry classes. This chemical information, curated by our expert scientists, is provided in alignment with our mission as a division of the American Chemical Society. | |
Explanation | The data from CAS Common Chemistry is provided under a CC-BY-NC 4.0 license, unless otherwise stated. | |
Record name | (4S,5S)-2,2-Dimethyl-1,3-dioxolane-4,5-dimethanol | |
Source | CAS Common Chemistry | |
URL | https://commonchemistry.cas.org/detail?cas_rn=50622-09-8 | |
Description | CAS Common Chemistry is an open community resource for accessing chemical information. Nearly 500,000 chemical substances from CAS REGISTRY cover areas of community interest, including common and frequently regulated chemicals, and those relevant to high school and undergraduate chemistry classes. This chemical information, curated by our expert scientists, is provided in alignment with our mission as a division of the American Chemical Society. | |
Explanation | The data from CAS Common Chemistry is provided under a CC-BY-NC 4.0 license, unless otherwise stated. | |
Record name | (2,2-Dimethyl-1,3-dioxolane-4,5-diyl)dimethanol | |
Source | EPA DSSTox | |
URL | https://comptox.epa.gov/dashboard/DTXSID40964859 | |
Description | DSSTox provides a high quality public chemistry resource for supporting improved predictive toxicology. | |
Record name | L-(+)-2,2-dimethyl-1,3-dioxolane-4,5-dimethanol | |
Source | European Chemicals Agency (ECHA) | |
URL | https://echa.europa.eu/substance-information/-/substanceinfo/100.051.490 | |
Description | The European Chemicals Agency (ECHA) is an agency of the European Union which is the driving force among regulatory authorities in implementing the EU's groundbreaking chemicals legislation for the benefit of human health and the environment as well as for innovation and competitiveness. | |
Explanation | Use of the information, documents and data from the ECHA website is subject to the terms and conditions of this Legal Notice, and subject to other binding limitations provided for under applicable law, the information, documents and data made available on the ECHA website may be reproduced, distributed and/or used, totally or in part, for non-commercial purposes provided that ECHA is acknowledged as the source: "Source: European Chemicals Agency, http://echa.europa.eu/". Such acknowledgement must be included in each copy of the material. ECHA permits and encourages organisations and individuals to create links to the ECHA website under the following cumulative conditions: Links can only be made to webpages that provide a link to the Legal Notice page. | |
Retrosynthesis Analysis
AI-Powered Synthesis Planning: Our tool employs the Template_relevance Pistachio, Template_relevance Bkms_metabolic, Template_relevance Pistachio_ringbreaker, Template_relevance Reaxys, Template_relevance Reaxys_biocatalysis model, leveraging a vast database of chemical reactions to predict feasible synthetic routes.
One-Step Synthesis Focus: Specifically designed for one-step synthesis, it provides concise and direct routes for your target compounds, streamlining the synthesis process.
Accurate Predictions: Utilizing the extensive PISTACHIO, BKMS_METABOLIC, PISTACHIO_RINGBREAKER, REAXYS, REAXYS_BIOCATALYSIS database, our tool offers high-accuracy predictions, reflecting the latest in chemical research and data.
Strategy Settings
Precursor scoring | Relevance Heuristic |
---|---|
Min. plausibility | 0.01 |
Model | Template_relevance |
Template Set | Pistachio/Bkms_metabolic/Pistachio_ringbreaker/Reaxys/Reaxys_biocatalysis |
Top-N result to add to graph | 6 |
Feasible Synthetic Routes
Q1: How is (+)-2,3-O-Isopropylidene-L-threitol used in the development of liquid crystals?
A1: this compound serves as a chiral building block for synthesizing compounds that can induce a cholesteric phase in nematic liquid crystals [, ]. By attaching mesogenic groups, such as oligophenylenevinylene (OPV) derivatives, to the this compound core, researchers can create chiral dopants. These dopants, when added to nematic liquid crystals, introduce helical twisting power (HTP), resulting in the formation of a cholesteric phase []. The alkylation of the OPV moiety further enhances the alignment within the nematic liquid crystal, promoting the appearance of the cholesteric phase [].
Q2: Can you provide examples of polymers synthesized using this compound and discuss their properties?
A2: Researchers have successfully synthesized polycarbonates [] and cyclocopolymers [] using this compound as a starting material.
Q3: Beyond liquid crystals, are there other applications of this compound in material science?
A3: Yes, this compound is a valuable monomer for creating bio-based polyesters []. For instance, incorporating it into poly(butylene succinate) (PBS) alongside isosorbide, another sugar-based diol, leads to improved mechanical properties and oxygen barrier performance compared to pure PBS []. This highlights the potential of this compound in developing sustainable and high-performance materials.
Q4: Has this compound been used in the synthesis of other chiral compounds?
A4: Yes, a chiral phosphoramidite ligand derived from this compound has been successfully employed in the rhodium-catalyzed hydrolytic cleavage of silacyclobutanes []. This reaction provides access to silanols, including Si-stereogenic silanols, with promising enantioselectivity [].
Disclaimer and Information on In-Vitro Research Products
Please be aware that all articles and product information presented on BenchChem are intended solely for informational purposes. The products available for purchase on BenchChem are specifically designed for in-vitro studies, which are conducted outside of living organisms. In-vitro studies, derived from the Latin term "in glass," involve experiments performed in controlled laboratory settings using cells or tissues. It is important to note that these products are not categorized as medicines or drugs, and they have not received approval from the FDA for the prevention, treatment, or cure of any medical condition, ailment, or disease. We must emphasize that any form of bodily introduction of these products into humans or animals is strictly prohibited by law. It is essential to adhere to these guidelines to ensure compliance with legal and ethical standards in research and experimentation.