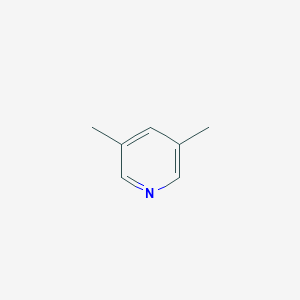
3,5-Dimethylpyridine
Overview
Description
3,5-Dimethylpyridine, also known as lutidine, is a derivative of pyridine with the chemical formula C7H9N . It is one of several dimethyl-substituted derivatives of pyridine .
Synthesis Analysis
The synthesis of 3,5-Dimethylpyridine has been explored using a two-step process via the synthesis of alkoxy-3,4-dihydro-2H-pyran as a precursor . The synthesis starts from methacrolein, propenyl ether, and 15N-labelled NH4Cl as a nitrogen source . Another method involves a three-component reaction of 3,5-diacetyl-2,6-dimethylpyridine, p-N-dimethylaminobenzaldehyde, and phenylhydrazine in an alcohol-alkaline medium .Molecular Structure Analysis
The molecular formula of 3,5-Dimethylpyridine is C7H9N . It is defined by the presence of a six-membered heterocyclic ring consisting of five carbon atoms and one nitrogen atom .Chemical Reactions Analysis
3,5-Dimethylpyridine can undergo various chemical reactions. For example, it can be hydrogenated to form 3,5-dimethylpiperidine . It can also participate in a three-component reaction to form bis(biarylhydrazone) derivatives .Physical And Chemical Properties Analysis
3,5-Dimethylpyridine has a density of 0.9±0.1 g/cm3, a boiling point of 170.7±9.0 °C at 760 mmHg, and a vapor pressure of 1.9±0.3 mmHg at 25°C . It has a molar refractivity of 34.0±0.3 cm3 and a molar volume of 115.2±3.0 cm3 .Scientific Research Applications
Synthesis of N labelled 3,5 dimethylpyridine
3,5-Dimethylpyridine is used in the synthesis of 15N-labelled pyridines , which are liquid and solid-state nuclear magnetic resonance (NMR) probes for chemical and biological environments . The 15N chemical shifts of these pyridines are sensitive to hydrogen-bond and protonation states . By varying the type and number of substituents, different target pyridines can be synthesized exhibiting different pKa values and molecular volumes .
Probing Chemical and Biological Environments
15N-labelled pyridines, including 3,5-dimethylpyridine, are important liquid and solid-state nuclear magnetic resonance (NMR) probes for chemical and biological environments . They have been used to explore the acidity of mesoporous surfaces or of biological environments using high-resolution solid-state NMR spectroscopy .
Monitoring Local H-bond and Protonation State
The 15N chemical shifts of 3,5-dimethylpyridine are very sensitive to the 15N1H distance and can be used to monitor the local H-bond and protonation state . This makes it a valuable tool in the study of chemical and biological environments .
Exploring Local Structures
The mobility of 3,5-dimethylpyridine to jump from one proton donor to another allows one to obtain interesting information about local structures . This makes it a useful tool in the study of molecular dynamics .
Thermodynamic Property Data
3,5-Dimethylpyridine is included in the collection of critically evaluated thermodynamic property data for pure compounds with a primary focus on organics . This data is generated through dynamic data analysis, as implemented in the NIST ThermoData Engine software package .
Catalyst Passivation Techniques
3,5-Dimethylpyridine has been used in the study of catalyst passivation techniques . The effectiveness of these techniques has been studied in situ with a magnetometer .
Mechanism of Action
Target of Action
3,5-Dimethylpyridine, also known as 3,5-Lutidine, is a heterocyclic organic compound It’s known that pyridines and their derivatives are often used as building blocks in the synthesis of various pharmaceuticals and agrochemicals .
Mode of Action
It’s known that pyridines can form hydrogen bonds due to their basicity . This property allows them to interact with various biological targets, potentially influencing their function.
Biochemical Pathways
It’s known that the biodegradation of pyridines proceeds via multiple pathways . Methylation, such as in 3,5-Dimethylpyridine, significantly retards the degradation of the pyridine ring , which could influence its interactions within biological systems.
Result of Action
It’s known that pyridines and their derivatives can have various biological effects depending on their specific structures and targets .
Action Environment
Environmental factors can influence the action, efficacy, and stability of 3,5-Dimethylpyridine. For instance, its degradation in the environment can be influenced by factors such as pH, temperature, and the presence of certain microorganisms
Safety and Hazards
properties
IUPAC Name |
3,5-dimethylpyridine | |
---|---|---|
Source | PubChem | |
URL | https://pubchem.ncbi.nlm.nih.gov | |
Description | Data deposited in or computed by PubChem | |
InChI |
InChI=1S/C7H9N/c1-6-3-7(2)5-8-4-6/h3-5H,1-2H3 | |
Source | PubChem | |
URL | https://pubchem.ncbi.nlm.nih.gov | |
Description | Data deposited in or computed by PubChem | |
InChI Key |
HWWYDZCSSYKIAD-UHFFFAOYSA-N | |
Source | PubChem | |
URL | https://pubchem.ncbi.nlm.nih.gov | |
Description | Data deposited in or computed by PubChem | |
Canonical SMILES |
CC1=CC(=CN=C1)C | |
Source | PubChem | |
URL | https://pubchem.ncbi.nlm.nih.gov | |
Description | Data deposited in or computed by PubChem | |
Molecular Formula |
C7H9N | |
Source | PubChem | |
URL | https://pubchem.ncbi.nlm.nih.gov | |
Description | Data deposited in or computed by PubChem | |
DSSTOX Substance ID |
DTXSID5052254 | |
Record name | 3,5-Dimethylpyridine | |
Source | EPA DSSTox | |
URL | https://comptox.epa.gov/dashboard/DTXSID5052254 | |
Description | DSSTox provides a high quality public chemistry resource for supporting improved predictive toxicology. | |
Molecular Weight |
107.15 g/mol | |
Source | PubChem | |
URL | https://pubchem.ncbi.nlm.nih.gov | |
Description | Data deposited in or computed by PubChem | |
Physical Description |
Liquid, Colorless liquid with an unpleasant odor; [Alfa Aesar MSDS] | |
Record name | Pyridine, 3,5-dimethyl- | |
Source | EPA Chemicals under the TSCA | |
URL | https://www.epa.gov/chemicals-under-tsca | |
Description | EPA Chemicals under the Toxic Substances Control Act (TSCA) collection contains information on chemicals and their regulations under TSCA, including non-confidential content from the TSCA Chemical Substance Inventory and Chemical Data Reporting. | |
Record name | 3,5-Dimethylpyridine | |
Source | Haz-Map, Information on Hazardous Chemicals and Occupational Diseases | |
URL | https://haz-map.com/Agents/17029 | |
Description | Haz-Map® is an occupational health database designed for health and safety professionals and for consumers seeking information about the adverse effects of workplace exposures to chemical and biological agents. | |
Explanation | Copyright (c) 2022 Haz-Map(R). All rights reserved. Unless otherwise indicated, all materials from Haz-Map are copyrighted by Haz-Map(R). No part of these materials, either text or image may be used for any purpose other than for personal use. Therefore, reproduction, modification, storage in a retrieval system or retransmission, in any form or by any means, electronic, mechanical or otherwise, for reasons other than personal use, is strictly prohibited without prior written permission. | |
Vapor Pressure |
1.74 [mmHg] | |
Record name | 3,5-Dimethylpyridine | |
Source | Haz-Map, Information on Hazardous Chemicals and Occupational Diseases | |
URL | https://haz-map.com/Agents/17029 | |
Description | Haz-Map® is an occupational health database designed for health and safety professionals and for consumers seeking information about the adverse effects of workplace exposures to chemical and biological agents. | |
Explanation | Copyright (c) 2022 Haz-Map(R). All rights reserved. Unless otherwise indicated, all materials from Haz-Map are copyrighted by Haz-Map(R). No part of these materials, either text or image may be used for any purpose other than for personal use. Therefore, reproduction, modification, storage in a retrieval system or retransmission, in any form or by any means, electronic, mechanical or otherwise, for reasons other than personal use, is strictly prohibited without prior written permission. | |
Product Name |
3,5-Dimethylpyridine | |
CAS RN |
591-22-0 | |
Record name | 3,5-Dimethylpyridine | |
Source | CAS Common Chemistry | |
URL | https://commonchemistry.cas.org/detail?cas_rn=591-22-0 | |
Description | CAS Common Chemistry is an open community resource for accessing chemical information. Nearly 500,000 chemical substances from CAS REGISTRY cover areas of community interest, including common and frequently regulated chemicals, and those relevant to high school and undergraduate chemistry classes. This chemical information, curated by our expert scientists, is provided in alignment with our mission as a division of the American Chemical Society. | |
Explanation | The data from CAS Common Chemistry is provided under a CC-BY-NC 4.0 license, unless otherwise stated. | |
Record name | 3,5-Dimethylpyridine | |
Source | ChemIDplus | |
URL | https://pubchem.ncbi.nlm.nih.gov/substance/?source=chemidplus&sourceid=0000591220 | |
Description | ChemIDplus is a free, web search system that provides access to the structure and nomenclature authority files used for the identification of chemical substances cited in National Library of Medicine (NLM) databases, including the TOXNET system. | |
Record name | 3,5-DIMETHYLPYRIDINE | |
Source | DTP/NCI | |
URL | https://dtp.cancer.gov/dtpstandard/servlet/dwindex?searchtype=NSC&outputformat=html&searchlist=60736 | |
Description | The NCI Development Therapeutics Program (DTP) provides services and resources to the academic and private-sector research communities worldwide to facilitate the discovery and development of new cancer therapeutic agents. | |
Explanation | Unless otherwise indicated, all text within NCI products is free of copyright and may be reused without our permission. Credit the National Cancer Institute as the source. | |
Record name | Pyridine, 3,5-dimethyl- | |
Source | EPA Chemicals under the TSCA | |
URL | https://www.epa.gov/chemicals-under-tsca | |
Description | EPA Chemicals under the Toxic Substances Control Act (TSCA) collection contains information on chemicals and their regulations under TSCA, including non-confidential content from the TSCA Chemical Substance Inventory and Chemical Data Reporting. | |
Record name | 3,5-Dimethylpyridine | |
Source | EPA DSSTox | |
URL | https://comptox.epa.gov/dashboard/DTXSID5052254 | |
Description | DSSTox provides a high quality public chemistry resource for supporting improved predictive toxicology. | |
Record name | 3,5-dimethylpyridine | |
Source | European Chemicals Agency (ECHA) | |
URL | https://echa.europa.eu/substance-information/-/substanceinfo/100.008.827 | |
Description | The European Chemicals Agency (ECHA) is an agency of the European Union which is the driving force among regulatory authorities in implementing the EU's groundbreaking chemicals legislation for the benefit of human health and the environment as well as for innovation and competitiveness. | |
Explanation | Use of the information, documents and data from the ECHA website is subject to the terms and conditions of this Legal Notice, and subject to other binding limitations provided for under applicable law, the information, documents and data made available on the ECHA website may be reproduced, distributed and/or used, totally or in part, for non-commercial purposes provided that ECHA is acknowledged as the source: "Source: European Chemicals Agency, http://echa.europa.eu/". Such acknowledgement must be included in each copy of the material. ECHA permits and encourages organisations and individuals to create links to the ECHA website under the following cumulative conditions: Links can only be made to webpages that provide a link to the Legal Notice page. | |
Record name | 3,5-DIMETHYLPYRIDINE | |
Source | FDA Global Substance Registration System (GSRS) | |
URL | https://gsrs.ncats.nih.gov/ginas/app/beta/substances/6Q4YPZ045V | |
Description | The FDA Global Substance Registration System (GSRS) enables the efficient and accurate exchange of information on what substances are in regulated products. Instead of relying on names, which vary across regulatory domains, countries, and regions, the GSRS knowledge base makes it possible for substances to be defined by standardized, scientific descriptions. | |
Explanation | Unless otherwise noted, the contents of the FDA website (www.fda.gov), both text and graphics, are not copyrighted. They are in the public domain and may be republished, reprinted and otherwise used freely by anyone without the need to obtain permission from FDA. Credit to the U.S. Food and Drug Administration as the source is appreciated but not required. | |
Synthesis routes and methods
Procedure details
Retrosynthesis Analysis
AI-Powered Synthesis Planning: Our tool employs the Template_relevance Pistachio, Template_relevance Bkms_metabolic, Template_relevance Pistachio_ringbreaker, Template_relevance Reaxys, Template_relevance Reaxys_biocatalysis model, leveraging a vast database of chemical reactions to predict feasible synthetic routes.
One-Step Synthesis Focus: Specifically designed for one-step synthesis, it provides concise and direct routes for your target compounds, streamlining the synthesis process.
Accurate Predictions: Utilizing the extensive PISTACHIO, BKMS_METABOLIC, PISTACHIO_RINGBREAKER, REAXYS, REAXYS_BIOCATALYSIS database, our tool offers high-accuracy predictions, reflecting the latest in chemical research and data.
Strategy Settings
Precursor scoring | Relevance Heuristic |
---|---|
Min. plausibility | 0.01 |
Model | Template_relevance |
Template Set | Pistachio/Bkms_metabolic/Pistachio_ringbreaker/Reaxys/Reaxys_biocatalysis |
Top-N result to add to graph | 6 |
Feasible Synthetic Routes
Q & A
ANone: 3,5-Dimethylpyridine has the molecular formula C₇H₉N and a molecular weight of 107.15 g/mol.
A: Researchers commonly utilize Infrared (IR) spectroscopy [, , , ], ¹H Nuclear Magnetic Resonance (¹H NMR) [, , , , ], and ¹³C Nuclear Magnetic Resonance (¹³C NMR) spectroscopy [, , ] to characterize 3,5-dimethylpyridine and its derivatives. ¹⁹F NMR is also employed for characterizing perfluoroalkyl derivatives []. Additionally, UV-Vis spectroscopy aids in understanding the electronic transitions within the molecule [, ].
A: Yes, 3,5-dimethylpyridine displays good solubility in various organic solvents like toluene [, , ], tetrahydrofuran [], pyridine [, ], and acetonitrile []. Its solubility in aromatic hydrocarbons is particularly notable [].
A: While not directly a catalyst, 3,5-dimethylpyridine acts as a ligand in various catalytic systems. For instance, a combination of (POCOPtBu)IrHCl, NaBArF4, and 3,5-dimethylpyridine catalyzes the regioselective silylation of the α-C(sp3)–H bond in 2-alkyl-1,3-azole derivatives [].
A: Yes, molecular orbital calculations have been used to determine the spin densities in 3,5-dimethylpyridine, providing insights into its electronic structure and reactivity []. Additionally, computational methods like NBO analysis have been applied to study the charge transfer characteristics of 3,5-dimethylpyridine during halogen bond formation [].
A: While specific formulation strategies are not extensively discussed in the provided research, forming complexes with metal ions, as seen in bis(O-butyldithiocarbonato)bis(3,5-dimethylpyridine)nickel(II) [], can influence solubility and stability. Researchers also explore the formation of salts and co-crystals with various organic molecules, like those involving phenol-based compounds, which can offer alternative formulation approaches for modifying physicochemical properties [].
A: Besides the spectroscopic methods mentioned earlier, X-ray crystallography plays a crucial role in determining the solid-state structures of 3,5-dimethylpyridine-containing compounds [, , , , , , ]. Additionally, thermogravimetric analysis (TGA) is used to assess the thermal stability of these compounds [, ].
A: 3,5-Dimethylpyridine readily coordinates to various metal centers, typically acting as a nitrogen-donor ligand. It forms complexes with transition metals like copper, manganese, nickel, and zinc [, , , , ]. These complexes exhibit diverse geometries, including square pyramidal [], octahedral [, ], and tetrahedral [, ] arrangements around the metal center.
ANone: 3,5-Dimethylpyridine can undergo a variety of chemical transformations, including:
- Halogenation: It can be brominated at the 2 and 6 positions, leading to the formation of 2,6-dibromo-3,5-dimethylpyridine [].
- N-oxidation: Reaction with hydrogen peroxide can yield 3,5-dimethylpyridine N-oxide, which serves as a versatile intermediate for further functionalization [, ].
- Nucleophilic Substitution: Perfluoro-3,5-dimethylpyridine undergoes nucleophilic substitution with ammonia, methoxide, and polyfluoroalkyl anions at the 2, 4, and 6 positions [].
A: 3,5-Dimethylpyridine has a rich history as a building block in coordination chemistry and material science. While not a pharmaceutical itself, it serves as a key intermediate in synthesizing important pharmaceutical compounds like omeprazole and esomeprazole, used in treating gastrointestinal disorders [, ].
Disclaimer and Information on In-Vitro Research Products
Please be aware that all articles and product information presented on BenchChem are intended solely for informational purposes. The products available for purchase on BenchChem are specifically designed for in-vitro studies, which are conducted outside of living organisms. In-vitro studies, derived from the Latin term "in glass," involve experiments performed in controlled laboratory settings using cells or tissues. It is important to note that these products are not categorized as medicines or drugs, and they have not received approval from the FDA for the prevention, treatment, or cure of any medical condition, ailment, or disease. We must emphasize that any form of bodily introduction of these products into humans or animals is strictly prohibited by law. It is essential to adhere to these guidelines to ensure compliance with legal and ethical standards in research and experimentation.