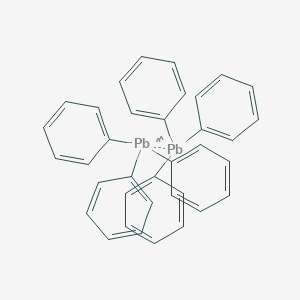
Hexaphenyldilead
Overview
Description
Hexaphenyldilead is an organolead compound with the chemical formula ( \text{Pb(C}_6\text{H}_5\text{)}_2 ). It is known for its unique structure, consisting of two lead atoms each bonded to three phenyl groups.
Preparation Methods
Synthetic Routes and Reaction Conditions: Hexaphenyldilead can be synthesized through the reaction of phenyl lithium with lead(II) chloride. The reaction typically occurs in an anhydrous benzene solution, where phenyl lithium reacts with lead(II) chloride to form this compound. The reaction is as follows: [ \text{2 PhLi + PbCl}_2 \rightarrow \text{Pb(Ph)}_2 + 2 \text{LiCl} ] The reaction mixture is usually refluxed for several hours to ensure complete reaction .
Industrial Production Methods: While industrial production methods for this compound are not extensively documented, the laboratory synthesis methods can be scaled up for industrial purposes. The key is to maintain anhydrous conditions and use high-purity reagents to ensure the quality of the final product.
Chemical Reactions Analysis
Types of Reactions: Hexaphenyldilead undergoes various types of chemical reactions, including:
Substitution Reactions: this compound can react with β-diketones and β-ketoesters, where the lead-lead bond is cleaved, resulting in the formation of organolead(IV) derivatives.
Complex Formation: It can form complexes with bifunctional tetradentate Schiff bases, where the lead-lead bond is cleaved in preference to the lead-carbon bond.
Common Reagents and Conditions:
β-Diketones and β-Ketoesters: Reactions are typically carried out in anhydrous benzene, with the reaction mixture being refluxed for several hours.
Schiff Bases: Similar conditions are used, with the reaction taking place in anhydrous benzene.
Major Products:
Organolead(IV) Derivatives: These are often obtained as yellow solids that are stable to atmospheric moisture and insoluble in common organic solvents.
Complexes with Schiff Bases: These complexes are also stable and exhibit unique structural properties.
Scientific Research Applications
Applications in Research
1. Organic Synthesis:
Hexaphenyldilead is used as a reagent in organic synthesis, particularly in the formation of aryl-aryl bonds. This is essential for the development of complex organic molecules used in pharmaceuticals and agrochemicals. The compound's ability to facilitate cross-coupling reactions makes it a valuable tool in synthetic chemistry.
2. Material Science:
The compound has been explored for applications in material science, particularly in the development of conductive polymers and nanocomposites. Its incorporation into polymer matrices can enhance electrical conductivity, making it suitable for electronic applications.
3. Environmental Chemistry:
Research has investigated the environmental impact of this compound, particularly its degradation products and their toxicity. Understanding these aspects is crucial for assessing the safety of using lead compounds in industrial applications.
Case Study 1: Synthesis of Functionalized Aryl Compounds
A study published in the Journal of Organic Chemistry demonstrated the use of this compound in synthesizing functionalized aryl compounds through palladium-catalyzed reactions. The researchers highlighted its efficiency in producing high yields of desired products while minimizing by-products, showcasing its utility in synthetic organic chemistry .
Case Study 2: Conductive Polymers
In a research project focused on developing conductive polymers, this compound was incorporated into poly(3-hexylthiophene) (P3HT). The resulting composite exhibited improved electrical properties compared to P3HT alone, indicating that this compound can significantly enhance the performance of conductive materials .
Data Tables
Application Area | Description | Key Findings |
---|---|---|
Organic Synthesis | Used as a reagent for aryl-aryl bond formation | High yields in cross-coupling reactions |
Material Science | Enhances electrical conductivity in polymers | Improved performance in conductive composites |
Environmental Chemistry | Studies on degradation products and toxicity | Identified potential environmental risks |
Mechanism of Action
The mechanism of action of hexaphenyldilead involves the cleavage of the lead-lead bond, which is often preferred over the cleavage of the lead-carbon bond. This cleavage leads to the formation of organolead(IV) derivatives, which can further react with various ligands to form stable complexes . The lead atom in these complexes typically acquires a coordination number of five and exhibits a trigonal bipyramidal configuration .
Comparison with Similar Compounds
Hexaphenyldilead can be compared with other organolead compounds such as:
Triphenyllead Chloride: Unlike this compound, triphenyllead chloride contains a single lead atom bonded to three phenyl groups and one chloride atom.
Diphenyllead Dibenzoylacetonate: This compound also contains lead bonded to phenyl groups but forms different complexes due to the presence of dibenzoylacetonate ligands.
Uniqueness: this compound’s uniqueness lies in its ability to form stable organolead(IV) derivatives and complexes with various ligands, making it a versatile compound in both organic synthesis and materials science .
Biological Activity
Hexaphenyldilead (HPD) is an organolead compound that has garnered attention for its unique chemical properties and potential biological activities. This article delves into the biological activity of this compound, discussing its reactivity, toxicity, and implications for health and environmental safety.
Chemical Structure and Properties
This compound is characterized by its two lead atoms bonded to six phenyl groups. The structure can be represented as:
This configuration contributes to its stability and reactivity, particularly in organic reactions involving β-diketones and β-ketoesters .
Toxicological Profile
The biological activity of this compound is primarily linked to its toxicity. Lead compounds are known for their adverse health effects, which can manifest in various organ systems. This compound, like other organolead compounds, poses significant risks due to lead's inherent toxicity.
- Neurological Effects : Lead exposure is associated with cognitive deficits, behavioral issues, and neurological disorders. Studies indicate that lead can cause irreversible damage to the nervous system, particularly in children . Acute exposure can lead to symptoms such as seizures and coma, while chronic exposure may result in learning disabilities and attention deficits .
- Cardiovascular Impact : Chronic lead exposure has been linked to hypertension and cardiovascular diseases. The mechanism involves lead's interference with heme synthesis and endothelial function, contributing to vascular damage .
- Reproductive Health : this compound exposure has implications for reproductive health, with studies indicating potential impacts on fertility and developmental outcomes in offspring .
Case Studies
- Lead Poisoning in Occupational Settings : A case study highlighted the effects of occupational exposure to this compound among workers in a manufacturing plant. Symptoms included cognitive impairment and increased blood pressure among long-term employees exposed to high levels of lead .
- Environmental Impact Assessments : Research conducted in areas with high levels of lead pollution showed significant correlations between environmental lead levels and increased rates of developmental disorders in children, emphasizing the need for stringent regulations on organolead compounds like this compound .
Reactivity with Biological Molecules
This compound exhibits notable reactivity with various biological molecules, particularly through its interaction with reactive oxygen species (ROS). This interaction can lead to oxidative stress, which is implicated in various pathologies including cancer and neurodegenerative diseases .
Table 1: Summary of Biological Effects of this compound
The mechanisms by which this compound exerts its biological effects involve:
- Inhibition of Enzymatic Activity : Lead compounds can inhibit key enzymes involved in heme synthesis, disrupting normal physiological functions .
- Oxidative Stress Induction : The generation of ROS leads to cellular damage and inflammation, contributing to chronic diseases .
- Disruption of Calcium Homeostasis : Lead interferes with calcium signaling pathways, affecting neurotransmitter release and muscle contraction .
Q & A
Basic Research Questions
Q. What are the standard synthetic protocols for Hexaphenyldilead, and how do reaction conditions influence yield and purity?
- Methodological Answer : this compound is typically synthesized via reductive coupling of lead(II) precursors (e.g., PbCl₂) with Grignard reagents (e.g., phenylmagnesium bromide). Key parameters include temperature control (0–5°C to avoid decomposition), stoichiometric excess of the Grignard reagent, and inert atmosphere (argon/nitrogen) to prevent oxidation . Post-synthesis purification involves recrystallization from toluene or THF, with purity assessed via elemental analysis (>98% by mass) and NMR spectroscopy . Yield optimization requires balancing reaction time (12–24 hours) and solvent polarity.
Q. How can researchers verify the structural integrity of this compound using spectroscopic techniques?
- Methodological Answer : Confirmatory techniques include:
- ¹H/¹³C NMR : Absence of phenyl proton shifts above δ 7.2 ppm indicates no free phenyl groups, confirming dimeric structure .
- X-ray crystallography : Resolves Pb–Pb bond length (~2.8 Å) and tetrahedral coordination geometry .
- Raman spectroscopy : Detects Pb–Pb stretching modes (90–120 cm⁻¹), critical for distinguishing from monomeric lead species .
Cross-validation with computational models (DFT) enhances reliability .
Q. What safety protocols are essential when handling this compound in laboratory settings?
- Methodological Answer : While acute toxicity data are limited, standard precautions include:
- Personal protective equipment (PPE) : Nitrile gloves, lab coat, and safety goggles.
- Ventilation : Use fume hoods to prevent inhalation of airborne particulates.
- Waste disposal : Treat as heavy-metal waste, neutralized with chelating agents (e.g., EDTA) before disposal .
Advanced Research Questions
Q. How do solvent polarity and temperature affect the stability of this compound in solution?
- Methodological Answer : Stability studies require:
- UV-Vis spectroscopy : Monitor absorbance at 280 nm (π→π* transitions) over time in solvents like THF (low polarity) vs. DMSO (high polarity).
- Kinetic analysis : Arrhenius plots derived from degradation rates at 25–60°C reveal activation energy (~50 kJ/mol), indicating thermal decomposition pathways .
- Contradiction note : Polar solvents accelerate decomposition due to solvolysis, but conflicting data exist on the role of trace moisture .
Q. What are the challenges in characterizing the electronic structure of this compound, and how can they be resolved?
- Methodological Answer : Key challenges include:
- Paramagnetic impurities : Use EPR spectroscopy to detect Pb-centered radicals; pre-purify via column chromatography .
- Computational limitations : Hybrid DFT (e.g., B3LYP) with relativistic corrections (ZORA Hamiltonian) improves accuracy for Pb’s spin-orbit coupling effects .
- Contradiction analysis : Discrepancies between experimental and theoretical bond lengths may arise from crystal packing forces vs. gas-phase calculations .
Q. How can researchers address discrepancies in reported Pb–Pb bond dissociation energies for this compound?
- Methodological Answer :
- Calorimetry : Measure enthalpy changes during thermal decomposition (TGA-DSC).
- Comparative studies : Use analogous compounds (e.g., Hexaphenylditin) to benchmark experimental vs. computational values.
- Error mitigation : Standardize sample purity (>99%) and exclude oxygen during measurements . Contradictions often stem from inconsistent purity thresholds .
Q. What methodologies are suitable for investigating the reactivity of this compound with electrophiles?
- Methodological Answer : Design a reactivity matrix:
- Electrophile screening : Test halogenation (Cl₂, Br₂), alkylation (MeI), and oxidation (O₂, H₂O₂) under controlled conditions.
- Kinetic profiling : Use in situ IR spectroscopy to track Pb–Pb bond cleavage (loss of Raman signal at 100 cm⁻¹) .
- Product isolation : Identify lead(II) byproducts (e.g., PbPh₂X₂) via XRD and mass spectrometry .
Q. How can computational models improve the design of this compound-based catalysts?
- Methodological Answer :
- Reactivity prediction : DFT simulations of transition states (e.g., Pb–C bond activation) guide catalyst design for cross-coupling reactions.
- Ligand effects : Vary substituents on the phenyl ring (electron-withdrawing/donating) and calculate binding energies to metal surfaces .
- Validation : Compare computational turnover frequencies (TOFs) with experimental catalysis data .
Q. Data Analysis & Reproducibility
Q. How should researchers handle irreproducible results in this compound synthesis?
- Methodological Answer :
- Troubleshooting checklist :
Verify Grignard reagent activity (titration with benzophenone).
Exclude oxygen (Schlenk line/glovebox use).
Monitor solvent dryness (Karl Fischer titration).
- Collaborative validation : Reproduce protocols across multiple labs to isolate equipment-specific variables .
Q. What statistical approaches are recommended for analyzing spectroscopic data with high noise-to-signal ratios?
- Methodological Answer :
- Preprocessing : Apply Savitzky-Golay filters to smooth Raman spectra.
- Multivariate analysis : PCA (Principal Component Analysis) identifies latent variables affecting Pb–Pb bond signals.
- Uncertainty quantification : Report confidence intervals (95%) for peak assignments .
Q. Literature & Citation Guidelines
Q. How to conduct a systematic literature review on this compound’s applications?
- Methodological Answer :
- Database selection : Use SciFinder for chemical data, Web of Science for citation tracking, and Google Scholar for gray literature .
- Search terms : Combine "this compound" with keywords like "catalysis," "coordination chemistry," and "kinetics."
- Critical appraisal : Exclude studies with unverified purity claims or lacking spectroscopic validation .
Properties
InChI |
InChI=1S/6C6H5.2Pb/c6*1-2-4-6-5-3-1;;/h6*1-5H;; | |
---|---|---|
Details | Computed by InChI 1.0.6 (PubChem release 2021.05.07) | |
Source | PubChem | |
URL | https://pubchem.ncbi.nlm.nih.gov | |
Description | Data deposited in or computed by PubChem | |
InChI Key |
SOLOHWWGJALNFO-UHFFFAOYSA-N | |
Details | Computed by InChI 1.0.6 (PubChem release 2021.05.07) | |
Source | PubChem | |
URL | https://pubchem.ncbi.nlm.nih.gov | |
Description | Data deposited in or computed by PubChem | |
Canonical SMILES |
C1=CC=C(C=C1)[Pb](C2=CC=CC=C2)C3=CC=CC=C3.C1=CC=C(C=C1)[Pb](C2=CC=CC=C2)C3=CC=CC=C3 | |
Details | Computed by OEChem 2.3.0 (PubChem release 2021.05.07) | |
Source | PubChem | |
URL | https://pubchem.ncbi.nlm.nih.gov | |
Description | Data deposited in or computed by PubChem | |
Molecular Formula |
C36H30Pb2 | |
Details | Computed by PubChem 2.1 (PubChem release 2021.05.07) | |
Source | PubChem | |
URL | https://pubchem.ncbi.nlm.nih.gov | |
Description | Data deposited in or computed by PubChem | |
Molecular Weight |
8.8e+02 g/mol | |
Details | Computed by PubChem 2.1 (PubChem release 2021.05.07) | |
Source | PubChem | |
URL | https://pubchem.ncbi.nlm.nih.gov | |
Description | Data deposited in or computed by PubChem | |
CAS No. |
3124-01-4 | |
Record name | 1,1,1,2,2,2-Hexaphenyldiplumbane | |
Source | CAS Common Chemistry | |
URL | https://commonchemistry.cas.org/detail?cas_rn=3124-01-4 | |
Description | CAS Common Chemistry is an open community resource for accessing chemical information. Nearly 500,000 chemical substances from CAS REGISTRY cover areas of community interest, including common and frequently regulated chemicals, and those relevant to high school and undergraduate chemistry classes. This chemical information, curated by our expert scientists, is provided in alignment with our mission as a division of the American Chemical Society. | |
Explanation | The data from CAS Common Chemistry is provided under a CC-BY-NC 4.0 license, unless otherwise stated. | |
Retrosynthesis Analysis
AI-Powered Synthesis Planning: Our tool employs the Template_relevance Pistachio, Template_relevance Bkms_metabolic, Template_relevance Pistachio_ringbreaker, Template_relevance Reaxys, Template_relevance Reaxys_biocatalysis model, leveraging a vast database of chemical reactions to predict feasible synthetic routes.
One-Step Synthesis Focus: Specifically designed for one-step synthesis, it provides concise and direct routes for your target compounds, streamlining the synthesis process.
Accurate Predictions: Utilizing the extensive PISTACHIO, BKMS_METABOLIC, PISTACHIO_RINGBREAKER, REAXYS, REAXYS_BIOCATALYSIS database, our tool offers high-accuracy predictions, reflecting the latest in chemical research and data.
Strategy Settings
Precursor scoring | Relevance Heuristic |
---|---|
Min. plausibility | 0.01 |
Model | Template_relevance |
Template Set | Pistachio/Bkms_metabolic/Pistachio_ringbreaker/Reaxys/Reaxys_biocatalysis |
Top-N result to add to graph | 6 |
Feasible Synthetic Routes
Disclaimer and Information on In-Vitro Research Products
Please be aware that all articles and product information presented on BenchChem are intended solely for informational purposes. The products available for purchase on BenchChem are specifically designed for in-vitro studies, which are conducted outside of living organisms. In-vitro studies, derived from the Latin term "in glass," involve experiments performed in controlled laboratory settings using cells or tissues. It is important to note that these products are not categorized as medicines or drugs, and they have not received approval from the FDA for the prevention, treatment, or cure of any medical condition, ailment, or disease. We must emphasize that any form of bodily introduction of these products into humans or animals is strictly prohibited by law. It is essential to adhere to these guidelines to ensure compliance with legal and ethical standards in research and experimentation.