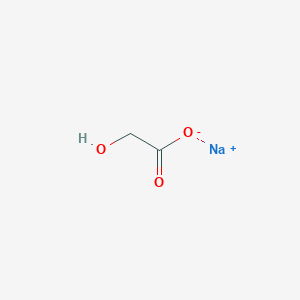
Sodium glycolate
Overview
Description
Sodium glycolate is a chemical compound with the molecular formula C₂H₃O₃Na. It is the sodium salt of glycolic acid, which is the simplest alpha hydroxy acid. This compound is known for its applications in various fields, including pharmaceuticals, cosmetics, and industrial processes.
Mechanism of Action
Target of Action
Sodium glycolate, the sodium salt of glycolic acid, is primarily used in the pharmaceutical industry as a disintegrant . Disintegrants are substances typically added to drug formulations that help the tablet disperse and dissolve when it comes into contact with moisture .
Mode of Action
The mode of action of this compound is based on its ability to absorb water quickly, leading to swelling which results in the rapid disintegration of tablets and granules . This rapid disintegration is crucial for immediate-release dosage forms, allowing the active pharmaceutical ingredient (API) to be released quickly into the body .
Biochemical Pathways
For instance, glycolic acid is a key intermediate in the glycolate pathway, a major pathway of photosynthesis in plants . .
Pharmacokinetics
Instead, it rapidly absorbs water, swells, and aids in the disintegration of the drug formulation, thereby facilitating the release of the API .
Result of Action
The primary result of this compound’s action is the rapid disintegration of pharmaceutical tablets and granules, which facilitates the release of the API into the body . This can lead to a faster onset of action for the drug, which can be particularly beneficial in the case of drugs where a rapid response is desired.
Action Environment
The action of this compound can be influenced by various environmental factors. For instance, the presence of moisture is crucial for this compound to absorb water and swell, thereby facilitating the disintegration of the drug formulation . Additionally, factors such as the pH of the environment, the presence of other excipients in the formulation, and the compression force used in tablet formation can also influence the effectiveness of this compound as a disintegrant .
Biochemical Analysis
Biochemical Properties
Sodium glycolate participates in various biochemical reactions. It is involved in the glycolate biosynthetic route, where key enzymes such as citrate synthase, isocitrate lyase, isocitrate dehydrogenase kinase/phosphatase, and glyoxylate reductase interact with it . The nature of these interactions involves the conversion of metabolic intermediates during the process of glycolate production .
Cellular Effects
This compound can influence various types of cells and cellular processes. For instance, in Escherichia coli, the overexpression of enzymes involved in the glycolate biosynthetic route leads to changes in cellular metabolism . This can impact cell function, including effects on cell signaling pathways, gene expression, and cellular metabolism .
Molecular Mechanism
The molecular mechanism of this compound involves its interaction with various biomolecules. For example, in the glycolate biosynthetic route, this compound interacts with enzymes such as citrate synthase and isocitrate lyase, leading to changes in enzyme activity and gene expression .
Temporal Effects in Laboratory Settings
In laboratory settings, the effects of this compound can change over time. For instance, in a study involving E. coli, the production of glycolate gradually increased with the growth of cells, reaching a maximum glycolate titer after a certain period . This indicates that this compound has a temporal effect on cellular function in in vitro studies .
Metabolic Pathways
This compound is involved in the glycolate metabolic pathway. In E. coli, for instance, it is part of the glycolate biosynthetic route, interacting with enzymes such as citrate synthase and isocitrate lyase . This involvement can affect metabolic flux and metabolite levels .
Preparation Methods
Synthetic Routes and Reaction Conditions: Sodium glycolate can be synthesized through the neutralization of glycolic acid with sodium hydroxide. The reaction is typically carried out in an aqueous solution, where glycolic acid (C₂H₄O₃) reacts with sodium hydroxide (NaOH) to form this compound (C₂H₃O₃Na) and water (H₂O):
C₂H₄O₃ + NaOH → C₂H₃O₃Na + H₂O
Industrial Production Methods: In industrial settings, this compound is produced by the same neutralization process but on a larger scale. The reaction conditions are optimized to ensure high yield and purity. The process involves careful control of temperature, pH, and concentration of reactants to achieve the desired product quality.
Chemical Reactions Analysis
Types of Reactions: Sodium glycolate undergoes various chemical reactions, including:
Oxidation: this compound can be oxidized to form oxalic acid and other by-products.
Reduction: It can be reduced to form ethylene glycol under specific conditions.
Substitution: this compound can participate in substitution reactions, where the sodium ion is replaced by other cations.
Common Reagents and Conditions:
Oxidation: Common oxidizing agents include potassium permanganate (KMnO₄) and hydrogen peroxide (H₂O₂).
Reduction: Reducing agents such as lithium aluminum hydride (LiAlH₄) can be used.
Substitution: Various metal salts can be used to replace the sodium ion.
Major Products Formed:
Oxidation: Oxalic acid (C₂H₂O₄)
Reduction: Ethylene glycol (C₂H₆O₂)
Substitution: Metal glycolates, depending on the substituting cation
Scientific Research Applications
Sodium glycolate has numerous applications in scientific research:
Chemistry: It is used as a reagent in organic synthesis and as a precursor for the synthesis of other compounds.
Biology: this compound is used in studies involving metabolic pathways and enzyme reactions.
Medicine: It is used in pharmaceutical formulations as a disintegrant and excipient.
Industry: this compound is used in the production of biodegradable polymers and as a corrosion inhibitor in various industrial processes.
Comparison with Similar Compounds
Sodium Lactate: Sodium lactate is the sodium salt of lactic acid and is used in similar applications, such as pharmaceuticals and cosmetics.
Sodium Citrate: Sodium citrate is the sodium salt of citric acid and is used as a buffering agent and preservative in various formulations.
Sodium Acetate: Sodium acetate is the sodium salt of acetic acid and is used in industrial processes and as a food additive.
Uniqueness of Sodium Glycolate: this compound is unique due to its specific properties as an alpha hydroxy acid salt. It has a distinct ability to act as an exfoliant in cosmetic formulations and as a disintegrant in pharmaceuticals, making it highly valuable in these industries.
Properties
CAS No. |
2836-32-0 |
---|---|
Molecular Formula |
C2H4NaO3 |
Molecular Weight |
99.04 g/mol |
IUPAC Name |
sodium;2-hydroxyacetate |
InChI |
InChI=1S/C2H4O3.Na/c3-1-2(4)5;/h3H,1H2,(H,4,5); |
InChI Key |
XPUJQEGMNQWMJI-UHFFFAOYSA-N |
Isomeric SMILES |
C(C(=O)[O-])O.[Na+] |
SMILES |
C(C(=O)[O-])O.[Na+] |
Canonical SMILES |
C(C(=O)O)O.[Na] |
2836-32-0 | |
physical_description |
Liquid |
Pictograms |
Corrosive; Irritant |
Related CAS |
79-14-1 (Parent) |
Synonyms |
glycolate glycolic acid glycolic acid, 1-(14)C-labeled glycolic acid, 2-(14)C-labeled glycolic acid, calcium salt glycolic acid, monoammonium salt glycolic acid, monolithium salt glycolic acid, monopotassium salt glycolic acid, monosodium salt glycolic acid, potassium salt hydroxyacetic acid potassium glycolate |
Origin of Product |
United States |
Synthesis routes and methods
Procedure details
Retrosynthesis Analysis
AI-Powered Synthesis Planning: Our tool employs the Template_relevance Pistachio, Template_relevance Bkms_metabolic, Template_relevance Pistachio_ringbreaker, Template_relevance Reaxys, Template_relevance Reaxys_biocatalysis model, leveraging a vast database of chemical reactions to predict feasible synthetic routes.
One-Step Synthesis Focus: Specifically designed for one-step synthesis, it provides concise and direct routes for your target compounds, streamlining the synthesis process.
Accurate Predictions: Utilizing the extensive PISTACHIO, BKMS_METABOLIC, PISTACHIO_RINGBREAKER, REAXYS, REAXYS_BIOCATALYSIS database, our tool offers high-accuracy predictions, reflecting the latest in chemical research and data.
Strategy Settings
Precursor scoring | Relevance Heuristic |
---|---|
Min. plausibility | 0.01 |
Model | Template_relevance |
Template Set | Pistachio/Bkms_metabolic/Pistachio_ringbreaker/Reaxys/Reaxys_biocatalysis |
Top-N result to add to graph | 6 |
Feasible Synthetic Routes
Q1: What is the molecular formula and weight of Sodium Glycolate?
A1: The molecular formula of this compound is C2H3NaO3, and its molecular weight is 98.03 g/mol. []
Q2: Is there any spectroscopic data available for this compound?
A2: Yes, researchers have conducted X-ray powder diffraction studies on this compound and refined its diffraction pattern and indices. The refined unit cell parameters for the orthorhombic system are: a = 1.0581(1) nm, b = 0.6440(1) nm, c = 0.5303(1) nm, V = 0.3613(1) nm3, Z = 4, Dx = 1.802 g·cm-3, Dm = 1.786 g·cm-3. []
Q3: What are some industrial applications of this compound?
A3: this compound is used in various industrial applications, including:
- Electrolyte in the production of Glycolic Acid: Electrodialysis using this compound as a starting material allows for the efficient and selective production of Glycolic Acid. []
- Food Additive: Carboxymethyl Cellulose (CMC) derived from palm-based sources can be produced using this compound. This CMC shows promise as a food additive. []
- Laundry Detergents: this compound is a component in laundry detergent formulations, particularly those containing highly water-soluble Carboxymethyl Cellulose particles. []
- Pharmaceutical Excipient: this compound is used as a disintegrant in pharmaceutical tablet formulations, facilitating rapid disintegration and drug release. [, , , ]
Q4: How does the concentration of Sodium Hydroxide impact Carboxymethyl Cellulose production using this compound?
A4: The concentration of Sodium Hydroxide significantly affects the properties of CMC produced using this compound. Higher alkali concentrations lead to the formation of this compound, which can reduce the purity of the resulting CMC. []
Q5: How does this compound contribute to tablet disintegration in pharmaceutical formulations?
A5: this compound, often in the form of Starch this compound, acts as a superdisintegrant in tablet formulations. It rapidly absorbs water and swells, creating pressure that breaks the tablet apart, facilitating faster drug release. [, , , ]
Q6: What is the role of this compound in Ethylene Glycol metabolism and toxicity?
A6: this compound is the sodium salt of Glycolic Acid, a key metabolite of Ethylene Glycol. While Ethylene Glycol itself has minimal toxicity, its metabolism to Glycolic Acid contributes significantly to its toxic effects. [, ]
Q7: Does this compound exhibit developmental toxicity in the absence of metabolic acidosis?
A7: Research suggests that this compound, even without inducing metabolic acidosis, can cause developmental toxicity. In rat studies, high doses of this compound led to decreased fetal body weight, skeletal variations, and fetal malformations. []
Q8: Does this compound influence enzyme activity in hyperoxaluric conditions?
A8: Studies indicate that this compound can modulate enzyme activity in hyperoxaluric conditions. In rat models, this compound administration led to significant changes in the activity of enzymes like Glycolic Acid Oxidase (GAO) and Lactate Dehydrogenase (LDH) in the liver. []
Q9: How does this compound affect sludge constituent solubility in nuclear waste management?
A9: Research at the Savannah River Site examined the impact of this compound on nuclear waste. Results indicate that this compound, at concentrations up to 10 g/L, did not significantly affect the solubility of major sludge constituents, including aluminum, sodium, and most salt anions. []
Q10: What is known about the environmental impact and degradation of this compound?
A10: While specific information on the environmental impact and degradation pathways of this compound might be limited in the provided research, it's crucial to consider its potential effects. As an organic salt, it might undergo biodegradation, but further research is needed to understand its fate and effects on ecosystems.
Q11: What are potential future research directions for this compound?
A11: Future research on this compound could focus on:
Disclaimer and Information on In-Vitro Research Products
Please be aware that all articles and product information presented on BenchChem are intended solely for informational purposes. The products available for purchase on BenchChem are specifically designed for in-vitro studies, which are conducted outside of living organisms. In-vitro studies, derived from the Latin term "in glass," involve experiments performed in controlled laboratory settings using cells or tissues. It is important to note that these products are not categorized as medicines or drugs, and they have not received approval from the FDA for the prevention, treatment, or cure of any medical condition, ailment, or disease. We must emphasize that any form of bodily introduction of these products into humans or animals is strictly prohibited by law. It is essential to adhere to these guidelines to ensure compliance with legal and ethical standards in research and experimentation.