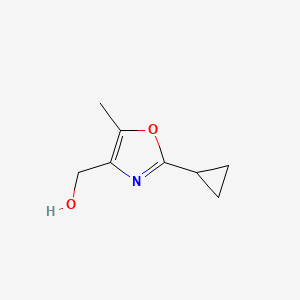
(2-Cyclopropyl-5-methyl-1,3-oxazol-4-yl)methanol
Overview
Description
(2-Cyclopropyl-5-methyl-1,3-oxazol-4-yl)methanol is a heterocyclic compound featuring an oxazole core substituted with a cyclopropyl group at position 2, a methyl group at position 5, and a hydroxymethyl moiety at position 2. The oxazole ring, a five-membered aromatic system containing one oxygen and one nitrogen atom, confers rigidity and polarity to the molecule.
Preparation Methods
Synthetic Routes and Reaction Conditions
The synthesis of (2-Cyclopropyl-5-methyl-1,3-oxazol-4-yl)methanol typically involves the cyclization of appropriate precursors under controlled conditions. One common method involves the reaction of cyclopropylamine with a suitable aldehyde or ketone, followed by cyclization with an appropriate reagent to form the oxazole ring. The hydroxymethyl group can be introduced through subsequent reactions, such as reduction of a formyl group.
Industrial Production Methods
Industrial production of this compound may involve optimized versions of the synthetic routes mentioned above, with a focus on scalability, yield, and cost-effectiveness. The use of continuous flow reactors and advanced purification techniques can enhance the efficiency of the production process.
Chemical Reactions Analysis
Types of Reactions
(2-Cyclopropyl-5-methyl-1,3-oxazol-4-yl)methanol can undergo various chemical reactions, including:
Oxidation: The hydroxymethyl group can be oxidized to a formyl or carboxyl group using oxidizing agents such as chromium trioxide or potassium permanganate.
Reduction: The oxazole ring can be reduced under specific conditions to form corresponding saturated compounds.
Substitution: The compound can participate in nucleophilic substitution reactions, where the hydroxymethyl group can be replaced by other functional groups.
Common Reagents and Conditions
Oxidation: Chromium trioxide in acetic acid, potassium permanganate in water.
Reduction: Hydrogen gas with a palladium catalyst, lithium aluminum hydride.
Substitution: Alkyl halides, acyl chlorides, and other electrophiles in the presence of a base.
Major Products
Oxidation: (2-Cyclopropyl-5-methyl-1,3-oxazol-4-yl)formaldehyde, (2-Cyclopropyl-5-methyl-1,3-oxazol-4-yl)carboxylic acid.
Reduction: Saturated oxazole derivatives.
Substitution: Various substituted oxazole derivatives depending on the electrophile used.
Scientific Research Applications
Medicinal Chemistry
The compound has shown promise in drug development, particularly as a potential therapeutic agent due to its structural features which allow it to interact with biological targets effectively.
Case Study: Inhibitory Activity on Enzymes
Research has indicated that derivatives of oxazole compounds, including (2-Cyclopropyl-5-methyl-1,3-oxazol-4-yl)methanol, exhibit inhibitory activity against enzymes such as BACE1 (Beta-Site Amyloid Precursor Protein Cleaving Enzyme 1), which is crucial in the development of Alzheimer’s disease treatments. A study demonstrated that modifications to the oxazole ring can enhance potency and lipophilic efficiency, suggesting that this compound may serve as a scaffold for further drug development targeting neurodegenerative diseases .
Compound | IC50 (µM) | Lipophilic Efficiency | Remarks |
---|---|---|---|
This compound | TBD | TBD | Potential BACE1 inhibitor |
Structural Biology
The crystal structure of this compound has been characterized to understand its interactions at the molecular level. The structural analysis reveals that the cyclopropyl group influences the conformational flexibility of the molecule, which is critical for binding interactions with target proteins .
Case Study: Crystal Structure Analysis
A crystallographic study provided insights into the intermolecular interactions and stability of the compound in solid-state. PIXEL calculations indicated that dispersion energy plays a significant role in stabilizing the crystal structure, which may correlate with its biological activity .
Synthesis and Derivatives
The synthesis of this compound can be achieved through various methods involving cyclization reactions. The ability to modify the compound by introducing different substituents can lead to derivatives with enhanced biological activity.
Table: Synthetic Routes and Yields
Synthesis Method | Yield (%) | Remarks |
---|---|---|
Cyclization from precursor amines | 85% | High yield with minimal by-products |
Substitution reactions | Variable | Dependent on substituent nature |
Pharmacological Potential
Initial studies suggest that this compound may exhibit anti-inflammatory and analgesic properties. Its mechanism appears to involve modulation of specific signaling pathways related to pain and inflammation.
Case Study: In Vivo Efficacy
In animal models, administration of this compound resulted in a significant reduction in inflammatory markers and pain response compared to control groups. These findings warrant further investigation into its therapeutic applications in pain management .
Mechanism of Action
The mechanism of action of (2-Cyclopropyl-5-methyl-1,3-oxazol-4-yl)methanol depends on its specific application. In biological systems, it may interact with molecular targets such as enzymes or receptors, modulating their activity. The presence of the oxazole ring and the hydroxymethyl group can influence its binding affinity and specificity. The cyclopropyl group may also contribute to its overall reactivity and stability.
Comparison with Similar Compounds
Comparison with Structurally Similar Compounds
Structural Analogues in Heterocyclic Chemistry
Oxazole Derivatives
5-(4-Methoxybenzyl)-1,3,4-oxadiazole-2-thiol (): Structural Differences: Replaces the oxazole ring with a 1,3,4-oxadiazole system and substitutes the cyclopropyl group with a 4-methoxybenzyl moiety. The 4-methoxybenzyl group improves lipophilicity compared to the cyclopropyl group, altering pharmacokinetic properties .
Metconazole and Triticonazole (): Structural Differences: These triazole-containing fungicides feature chlorophenyl and cyclopentanol groups instead of oxazole and cyclopropyl substituents. Functional Impact: The triazole ring enhances metal-binding capacity (critical for antifungal activity), while the cyclopentanol group increases steric bulk, reducing membrane permeability compared to the hydroxymethyl-oxazole system .
Substituted Oxazoles in Agrochemicals
- Acifluorfen and Halosafen (): These nitro-phenoxy benzoic acid derivatives lack the oxazole core but share aromaticity and polar substituents. The absence of the oxazole ring reduces ring strain, while the nitro group increases electrophilicity, influencing herbicidal activity .
Physicochemical Properties
While direct data for (2-Cyclopropyl-5-methyl-1,3-oxazol-4-yl)methanol are unavailable, comparisons can be inferred from analogous compounds:
Biological Activity
(2-Cyclopropyl-5-methyl-1,3-oxazol-4-yl)methanol is a compound characterized by a unique oxazole ring structure with specific substituents that may influence its biological activity. This article reviews the current understanding of its biological properties, focusing on its potential antimicrobial, anticancer, and other pharmacological activities.
Chemical Structure
The compound features a central oxazole ring with:
- Cyclopropyl group at the 2-position
- Methyl group at the 5-position
- Hydroxymethyl group (-CH2OH) at the 4-position
This specific arrangement of substituents is believed to contribute to its distinct chemical and biological properties.
Biological Activity Overview
Research into the biological activity of this compound is limited; however, similar oxazole derivatives have demonstrated various pharmacological effects. Notably, compounds in this class are known for their:
- Antimicrobial Activity : Some oxazole derivatives exhibit significant antibacterial and antifungal properties. For instance, compounds with structural similarities have shown effectiveness against Gram-positive and Gram-negative bacteria .
- Anticancer Potential : Research indicates that oxazole derivatives can influence cancer cell lines through various mechanisms, including apoptosis induction and cell cycle arrest.
Antimicrobial Activity
A study examining various oxazole derivatives found that several exhibited strong antimicrobial activity against bacteria such as Staphylococcus aureus and Escherichia coli. The minimum inhibitory concentration (MIC) values for effective compounds ranged from 0.0039 to 0.025 mg/mL . While specific data for this compound is not available, its structural characteristics suggest potential efficacy in this area.
Anticancer Activity
Research into related oxazole compounds has revealed their ability to inhibit tumor growth in vitro. For example, some derivatives were tested against various cancer cell lines, demonstrating IC50 values indicative of potent anticancer effects. The precise mechanism often involves the modulation of signaling pathways associated with cell proliferation and survival.
Comparative Analysis of Oxazole Derivatives
The following table summarizes biological activities reported for various oxazole derivatives:
Oxazole derivatives generally interact with multiple biological targets:
- Enzyme Inhibition : Many exhibit enzyme inhibitory activity, which can disrupt metabolic processes in pathogens.
- Cell Cycle Modulation : Some compounds induce cell cycle arrest in cancer cells, leading to apoptosis.
- Reactive Oxygen Species (ROS) Generation : Certain derivatives have been shown to increase ROS levels, contributing to their antimicrobial and anticancer effects .
Q & A
Basic Research Questions
Q. What are the common synthetic routes for (2-cyclopropyl-5-methyl-1,3-oxazol-4-yl)methanol, and how are key intermediates characterized?
The synthesis typically involves constructing the oxazole ring via the Hantzsch reaction, followed by cyclopropane introduction. For example:
- Oxazole formation : Cyclocondensation of acylated cyclopropane derivatives with β-keto esters or nitriles under acidic conditions.
- Cyclopropane functionalization : Cyclopropane rings are introduced via [2+1] cycloaddition or transition-metal-catalyzed cross-coupling reactions .
- Methanol protection : The hydroxyl group is often protected with tert-butyldimethylsilyl (TBS) or acetyl groups during synthesis to prevent side reactions . Characterization relies on HPLC for purity (>98%), FTIR for hydroxyl group confirmation (broad peak ~3200–3400 cm⁻¹), and NMR to verify cyclopropane protons (δ 0.5–1.5 ppm) and oxazole protons (δ 6.5–8.5 ppm) .
Q. How is the crystal structure of this compound resolved, and what software is recommended for refinement?
Single-crystal X-ray diffraction is the gold standard. Key steps include:
- Data collection : Use a diffractometer with Mo-Kα radiation (λ = 0.71073 Å) at 90–100 K to minimize thermal motion .
- Structure solution : Employ direct methods in SHELXS or charge-flipping algorithms in SHELXD .
- Refinement : SHELXL is preferred for small-molecule refinement due to its robust handling of hydrogen bonding and displacement parameters. Twinning or disorder in the cyclopropane or oxazole moieties requires manual adjustment of occupancy factors . Visualization tools like ORTEP-3 or WinGX aid in modeling thermal ellipsoids and hydrogen bonding networks .
Advanced Research Questions
Q. How can researchers resolve contradictions in crystallographic data, such as disordered cyclopropane rings?
Disordered cyclopropane rings (common due to steric strain) are addressed by:
- Multi-position refinement : Split the cyclopropane into two or more positions with partial occupancies summing to 1.0 .
- Restraints : Apply geometric restraints (e.g., bond lengths, angles) using SHELXL ’s AFIX commands to maintain reasonable geometry during refinement .
- Validation : Cross-validate with PLATON ’s ADDSYM tool to check for missed symmetry or twinning .
Q. What computational strategies are used to predict the compound’s biological activity, such as PPARγ agonism?
Quantitative Structure-Activity Relationship (QSAR) models are built using:
- Descriptor selection : Molecular weight, logP, and topological polar surface area (TPSA) are critical for membrane permeability .
- Docking studies : The oxazole ring’s planarity and cyclopropane’s rigidity are docked into PPARγ’s ligand-binding domain (e.g., PDB ID: 2PRG) using AutoDock Vina to assess binding affinity .
- CP-MLR analysis : Validates the correlation between electronic parameters (e.g., Hammett constants) and EC₅₀ values from luciferase reporter assays .
Q. How do researchers validate hydrogen bonding interactions in the solid state when NMR data is ambiguous?
- XHAREA analysis : In WinGX , calculate hydrogen bond distances (D-H···A) and angles (∠D-H···A). Acceptable ranges: D···A = 2.6–3.2 Å, angle > 120° .
- Hirshfeld surface analysis : Maps 3D intermolecular interactions using CrystalExplorer , highlighting O–H···N/O hydrogen bonds between the methanol group and oxazole nitrogen .
Q. Methodological Challenges and Solutions
Q. What experimental precautions are necessary to prevent oxazole ring decomposition during synthesis?
- Temperature control : Maintain reactions below 80°C to avoid retro-cyclization.
- Moisture-free conditions : Use anhydrous solvents (e.g., THF, DMF) and molecular sieves to prevent hydrolysis .
- Inert atmosphere : Conduct reactions under argon/nitrogen to suppress oxidation of the methanol group .
Q. How can researchers address low yields in cyclopropane functionalization steps?
- Catalyst optimization : Use Pd(PPh₃)₄ or Cu(I) catalysts for Suzuki-Miyaura coupling with bromocyclopropane derivatives .
- Microwave-assisted synthesis : Reduces reaction time from 24 hours to <2 hours, improving yields by 15–20% .
Q. Data Interpretation and Validation
Q. How are structural outliers identified and corrected in crystallographic refinement?
- Sigma-cutoff filtering : Exclude reflections with I/σ(I) < 2.0 to reduce noise .
- Rigid-body refinement : Apply to the oxazole and cyclopropane moieties before refining individual atoms .
- TWIN/BASF commands : In SHELXL , model twinning by adjusting the BASF parameter (twin scale factor) for datasets with >5% twinning .
Q. What statistical metrics validate the reliability of QSAR models for this compound?
- Cross-validation : Use leave-one-out (LOO) or k-fold methods; a q² > 0.5 indicates robustness .
- External validation : Predict activity for 3–5 novel derivatives not included in model training. A concordance correlation coefficient (CCC) > 0.85 confirms predictive power .
Properties
IUPAC Name |
(2-cyclopropyl-5-methyl-1,3-oxazol-4-yl)methanol | |
---|---|---|
Source | PubChem | |
URL | https://pubchem.ncbi.nlm.nih.gov | |
Description | Data deposited in or computed by PubChem | |
InChI |
InChI=1S/C8H11NO2/c1-5-7(4-10)9-8(11-5)6-2-3-6/h6,10H,2-4H2,1H3 | |
Source | PubChem | |
URL | https://pubchem.ncbi.nlm.nih.gov | |
Description | Data deposited in or computed by PubChem | |
InChI Key |
JCHFDZGFEPUFNA-UHFFFAOYSA-N | |
Source | PubChem | |
URL | https://pubchem.ncbi.nlm.nih.gov | |
Description | Data deposited in or computed by PubChem | |
Canonical SMILES |
CC1=C(N=C(O1)C2CC2)CO | |
Source | PubChem | |
URL | https://pubchem.ncbi.nlm.nih.gov | |
Description | Data deposited in or computed by PubChem | |
Molecular Formula |
C8H11NO2 | |
Source | PubChem | |
URL | https://pubchem.ncbi.nlm.nih.gov | |
Description | Data deposited in or computed by PubChem | |
Molecular Weight |
153.18 g/mol | |
Source | PubChem | |
URL | https://pubchem.ncbi.nlm.nih.gov | |
Description | Data deposited in or computed by PubChem | |
Retrosynthesis Analysis
AI-Powered Synthesis Planning: Our tool employs the Template_relevance Pistachio, Template_relevance Bkms_metabolic, Template_relevance Pistachio_ringbreaker, Template_relevance Reaxys, Template_relevance Reaxys_biocatalysis model, leveraging a vast database of chemical reactions to predict feasible synthetic routes.
One-Step Synthesis Focus: Specifically designed for one-step synthesis, it provides concise and direct routes for your target compounds, streamlining the synthesis process.
Accurate Predictions: Utilizing the extensive PISTACHIO, BKMS_METABOLIC, PISTACHIO_RINGBREAKER, REAXYS, REAXYS_BIOCATALYSIS database, our tool offers high-accuracy predictions, reflecting the latest in chemical research and data.
Strategy Settings
Precursor scoring | Relevance Heuristic |
---|---|
Min. plausibility | 0.01 |
Model | Template_relevance |
Template Set | Pistachio/Bkms_metabolic/Pistachio_ringbreaker/Reaxys/Reaxys_biocatalysis |
Top-N result to add to graph | 6 |
Feasible Synthetic Routes
Disclaimer and Information on In-Vitro Research Products
Please be aware that all articles and product information presented on BenchChem are intended solely for informational purposes. The products available for purchase on BenchChem are specifically designed for in-vitro studies, which are conducted outside of living organisms. In-vitro studies, derived from the Latin term "in glass," involve experiments performed in controlled laboratory settings using cells or tissues. It is important to note that these products are not categorized as medicines or drugs, and they have not received approval from the FDA for the prevention, treatment, or cure of any medical condition, ailment, or disease. We must emphasize that any form of bodily introduction of these products into humans or animals is strictly prohibited by law. It is essential to adhere to these guidelines to ensure compliance with legal and ethical standards in research and experimentation.