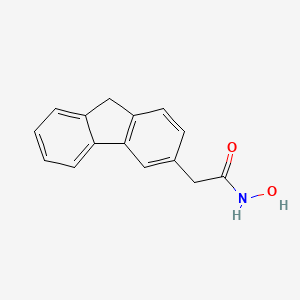
9H-Fluorene-3-acetamide, N-hydroxy-
- Click on QUICK INQUIRY to receive a quote from our team of experts.
- With the quality product at a COMPETITIVE price, you can focus more on your research.
Overview
Description
9H-Fluorene-3-acetamide, N-hydroxy- is a chemical compound with the molecular formula C15H13NO2 and a molecular weight of 239.2692 g/mol . This compound is known for its unique structure, which includes a fluorene backbone with an acetamide group and a hydroxyl group attached. It is also referred to by other names such as N-Hydroxy-2-acetamidofluorene and N-Hydroxy-2-acetaminofluorene .
Preparation Methods
The synthesis of 9H-Fluorene-3-acetamide, N-hydroxy- can be achieved through various synthetic routes. One common method involves the reaction of 9H-fluorene with acetic anhydride in the presence of a catalyst to form 9H-fluorene-3-acetamide. This intermediate is then hydroxylated using hydroxylamine hydrochloride under basic conditions to yield the final product . Industrial production methods may involve similar steps but are optimized for higher yields and purity.
Chemical Reactions Analysis
9H-Fluorene-3-acetamide, N-hydroxy- undergoes several types of chemical reactions, including:
Oxidation: The compound can be oxidized to form 9-fluorenone derivatives.
Reduction: Reduction reactions can convert the compound into various hydroxyfluorene derivatives.
Common reagents used in these reactions include oxidizing agents like potassium permanganate and reducing agents such as sodium borohydride. The major products formed from these reactions depend on the specific conditions and reagents used.
Scientific Research Applications
9H-Fluorene-3-acetamide, N-hydroxy- has several scientific research applications:
Mechanism of Action
The mechanism of action of 9H-Fluorene-3-acetamide, N-hydroxy- involves its interaction with specific molecular targets and pathways. The hydroxyl group can form hydrogen bonds with various biomolecules, influencing their structure and function. Additionally, the acetamide group can participate in nucleophilic and electrophilic reactions, affecting the compound’s reactivity and interactions .
Comparison with Similar Compounds
9H-Fluorene-3-acetamide, N-hydroxy- can be compared with other similar compounds such as:
N-Hydroxy-2-acetamidofluorene: Similar structure but different functional groups.
N-Hydroxy-2-acetaminofluorene: Another closely related compound with slight variations in its chemical structure.
These compounds share similar properties but differ in their specific reactivity and applications, highlighting the uniqueness of 9H-Fluorene-3-acetamide, N-hydroxy-.
Properties
CAS No. |
31339-04-5 |
---|---|
Molecular Formula |
C15H13NO2 |
Molecular Weight |
239.27 g/mol |
IUPAC Name |
2-(9H-fluoren-3-yl)-N-hydroxyacetamide |
InChI |
InChI=1S/C15H13NO2/c17-15(16-18)8-10-5-6-12-9-11-3-1-2-4-13(11)14(12)7-10/h1-7,18H,8-9H2,(H,16,17) |
InChI Key |
VVHSFDYCBPHPHT-UHFFFAOYSA-N |
Canonical SMILES |
C1C2=C(C=C(C=C2)CC(=O)NO)C3=CC=CC=C31 |
Origin of Product |
United States |
Disclaimer and Information on In-Vitro Research Products
Please be aware that all articles and product information presented on BenchChem are intended solely for informational purposes. The products available for purchase on BenchChem are specifically designed for in-vitro studies, which are conducted outside of living organisms. In-vitro studies, derived from the Latin term "in glass," involve experiments performed in controlled laboratory settings using cells or tissues. It is important to note that these products are not categorized as medicines or drugs, and they have not received approval from the FDA for the prevention, treatment, or cure of any medical condition, ailment, or disease. We must emphasize that any form of bodily introduction of these products into humans or animals is strictly prohibited by law. It is essential to adhere to these guidelines to ensure compliance with legal and ethical standards in research and experimentation.