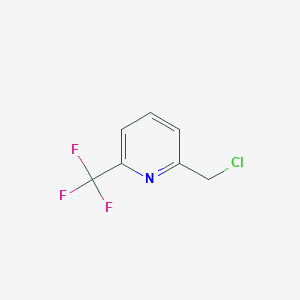
2-(Chloromethyl)-6-(trifluoromethyl)pyridine
Overview
Description
2-(Chloromethyl)-6-(trifluoromethyl)pyridine is a useful research compound. Its molecular formula is C7H5ClF3N and its molecular weight is 195.57 g/mol. The purity is usually 95%.
BenchChem offers high-quality this compound suitable for many research applications. Different packaging options are available to accommodate customers' requirements. Please inquire for more information about this compound including the price, delivery time, and more detailed information at info@benchchem.com.
Mechanism of Action
Target of Action
The trifluoromethyl group, a component of this compound, plays an increasingly important role in pharmaceuticals, agrochemicals, and materials .
Mode of Action
Compounds with a trifluoromethyl group have been associated with the trifluoromethylation of carbon-centered radical intermediates . This suggests that 2-(Chloromethyl)-6-(trifluoromethyl)pyridine may interact with its targets through a radical mechanism.
Biochemical Pathways
The trifluoromethylation process, which this compound may be involved in, can affect various biochemical pathways .
Result of Action
The trifluoromethyl group’s involvement in various pharmaceuticals, agrochemicals, and materials suggests that it may have significant effects at the molecular and cellular levels .
Biochemical Analysis
Biochemical Properties
2-(Chloromethyl)-6-(trifluoromethyl)pyridine plays a crucial role in biochemical reactions due to its ability to interact with a variety of enzymes, proteins, and other biomolecules. The presence of the chloromethyl group allows it to form covalent bonds with nucleophilic sites on proteins and enzymes, potentially leading to enzyme inhibition or activation. Additionally, the trifluoromethyl group can enhance the compound’s lipophilicity, facilitating its interaction with hydrophobic pockets in proteins. Studies have shown that this compound can interact with cytochrome P450 enzymes, leading to alterations in their catalytic activity .
Cellular Effects
The effects of this compound on various types of cells and cellular processes are profound. This compound has been observed to influence cell signaling pathways, gene expression, and cellular metabolism. For instance, it can modulate the activity of kinases and phosphatases, leading to changes in phosphorylation states of key signaling proteins. Additionally, this compound can affect the expression of genes involved in metabolic pathways, thereby altering cellular metabolism . These effects can result in changes in cell proliferation, differentiation, and apoptosis.
Molecular Mechanism
At the molecular level, this compound exerts its effects through several mechanisms. One of the primary mechanisms involves the formation of covalent bonds with nucleophilic residues in enzymes, leading to enzyme inhibition or activation. This compound can also bind to specific receptors or transporters on the cell surface, triggering downstream signaling cascades. Furthermore, this compound can influence gene expression by interacting with transcription factors or epigenetic modifiers . These interactions can lead to changes in the transcriptional landscape of the cell, ultimately affecting cellular function.
Temporal Effects in Laboratory Settings
The temporal effects of this compound in laboratory settings are influenced by its stability and degradation over time. Studies have shown that this compound is relatively stable under standard laboratory conditions, but it can undergo hydrolysis or oxidation over extended periods . The long-term effects of this compound on cellular function have been investigated in both in vitro and in vivo studies. These studies indicate that prolonged exposure to the compound can lead to sustained changes in cellular metabolism and gene expression, potentially resulting in altered cell behavior.
Dosage Effects in Animal Models
The effects of this compound vary with different dosages in animal models. At low doses, the compound may exhibit minimal toxicity and primarily modulate specific biochemical pathways. At higher doses, this compound can induce toxic effects, including oxidative stress, inflammation, and cell death . Threshold effects have been observed, where a certain dosage level is required to elicit significant biochemical and cellular responses. These findings highlight the importance of dosage optimization in experimental and therapeutic applications.
Metabolic Pathways
This compound is involved in several metabolic pathways, interacting with various enzymes and cofactors. The compound can be metabolized by cytochrome P450 enzymes, leading to the formation of reactive intermediates that can further interact with cellular components . Additionally, this compound can influence metabolic flux by modulating the activity of key metabolic enzymes, resulting in changes in metabolite levels and overall cellular metabolism.
Transport and Distribution
The transport and distribution of this compound within cells and tissues are mediated by specific transporters and binding proteins. The compound’s lipophilicity, conferred by the trifluoromethyl group, facilitates its diffusion across cell membranes and accumulation in hydrophobic compartments . Additionally, this compound can interact with transporters such as ABC transporters, influencing its cellular uptake and distribution.
Subcellular Localization
The subcellular localization of this compound is determined by its interactions with targeting signals and post-translational modifications. The compound can localize to specific cellular compartments, such as the endoplasmic reticulum, mitochondria, or nucleus, depending on its binding partners and modifications . This localization can impact the compound’s activity and function, as it may interact with different biomolecules in distinct cellular environments.
Properties
IUPAC Name |
2-(chloromethyl)-6-(trifluoromethyl)pyridine | |
---|---|---|
Source | PubChem | |
URL | https://pubchem.ncbi.nlm.nih.gov | |
Description | Data deposited in or computed by PubChem | |
InChI |
InChI=1S/C7H5ClF3N/c8-4-5-2-1-3-6(12-5)7(9,10)11/h1-3H,4H2 | |
Source | PubChem | |
URL | https://pubchem.ncbi.nlm.nih.gov | |
Description | Data deposited in or computed by PubChem | |
InChI Key |
IZCYKFUHFBLPJN-UHFFFAOYSA-N | |
Source | PubChem | |
URL | https://pubchem.ncbi.nlm.nih.gov | |
Description | Data deposited in or computed by PubChem | |
Canonical SMILES |
C1=CC(=NC(=C1)C(F)(F)F)CCl | |
Source | PubChem | |
URL | https://pubchem.ncbi.nlm.nih.gov | |
Description | Data deposited in or computed by PubChem | |
Molecular Formula |
C7H5ClF3N | |
Source | PubChem | |
URL | https://pubchem.ncbi.nlm.nih.gov | |
Description | Data deposited in or computed by PubChem | |
DSSTOX Substance ID |
DTXSID40737141 | |
Record name | 2-(Chloromethyl)-6-(trifluoromethyl)pyridine | |
Source | EPA DSSTox | |
URL | https://comptox.epa.gov/dashboard/DTXSID40737141 | |
Description | DSSTox provides a high quality public chemistry resource for supporting improved predictive toxicology. | |
Molecular Weight |
195.57 g/mol | |
Source | PubChem | |
URL | https://pubchem.ncbi.nlm.nih.gov | |
Description | Data deposited in or computed by PubChem | |
CAS No. |
849094-03-7 | |
Record name | 2-(Chloromethyl)-6-(trifluoromethyl)pyridine | |
Source | EPA DSSTox | |
URL | https://comptox.epa.gov/dashboard/DTXSID40737141 | |
Description | DSSTox provides a high quality public chemistry resource for supporting improved predictive toxicology. | |
Synthesis routes and methods
Procedure details
Retrosynthesis Analysis
AI-Powered Synthesis Planning: Our tool employs the Template_relevance Pistachio, Template_relevance Bkms_metabolic, Template_relevance Pistachio_ringbreaker, Template_relevance Reaxys, Template_relevance Reaxys_biocatalysis model, leveraging a vast database of chemical reactions to predict feasible synthetic routes.
One-Step Synthesis Focus: Specifically designed for one-step synthesis, it provides concise and direct routes for your target compounds, streamlining the synthesis process.
Accurate Predictions: Utilizing the extensive PISTACHIO, BKMS_METABOLIC, PISTACHIO_RINGBREAKER, REAXYS, REAXYS_BIOCATALYSIS database, our tool offers high-accuracy predictions, reflecting the latest in chemical research and data.
Strategy Settings
Precursor scoring | Relevance Heuristic |
---|---|
Min. plausibility | 0.01 |
Model | Template_relevance |
Template Set | Pistachio/Bkms_metabolic/Pistachio_ringbreaker/Reaxys/Reaxys_biocatalysis |
Top-N result to add to graph | 6 |
Feasible Synthetic Routes
Disclaimer and Information on In-Vitro Research Products
Please be aware that all articles and product information presented on BenchChem are intended solely for informational purposes. The products available for purchase on BenchChem are specifically designed for in-vitro studies, which are conducted outside of living organisms. In-vitro studies, derived from the Latin term "in glass," involve experiments performed in controlled laboratory settings using cells or tissues. It is important to note that these products are not categorized as medicines or drugs, and they have not received approval from the FDA for the prevention, treatment, or cure of any medical condition, ailment, or disease. We must emphasize that any form of bodily introduction of these products into humans or animals is strictly prohibited by law. It is essential to adhere to these guidelines to ensure compliance with legal and ethical standards in research and experimentation.