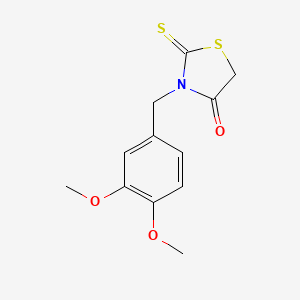
Rhodanine, 3-veratryl-
- Click on QUICK INQUIRY to receive a quote from our team of experts.
- With the quality product at a COMPETITIVE price, you can focus more on your research.
Overview
Description
Rhodanine, 3-veratryl- is a derivative of rhodanine, a five-membered heterocyclic compound containing a thiazole nucleus with a thioxo group on the second carbon and a carbonyl group on the fourth carbon . Rhodanine derivatives have garnered significant attention due to their broad spectrum of pharmacological activities, including antidiabetic, antibacterial, antifungal, and anticancer properties .
Preparation Methods
Synthetic Routes and Reaction Conditions
Rhodanine derivatives, including Rhodanine, 3-veratryl-, can be synthesized through various methods. One common approach involves the reaction of rhodanine with aromatic aldehydes under basic conditions to form N-substituted rhodanines . Another method involves the use of Schiff bases, where rhodanine reacts with aromatic aldehydes in a two-step reaction . These reactions typically occur at room temperature and can be performed in aqueous media, making them environmentally friendly .
Industrial Production Methods
Industrial production of rhodanine derivatives often involves multi-step synthesis processes. These methods require highly reactive functional groups and smooth reaction conditions to ensure high yields and purity . The use of catalysts and optimized reaction conditions can further enhance the efficiency of the synthesis process.
Chemical Reactions Analysis
Types of Reactions
Rhodanine, 3-veratryl-, like other rhodanine derivatives, undergoes various chemical reactions, including:
Oxidation: Rhodanine derivatives can be oxidized to form sulfoxides and sulfones.
Reduction: Reduction reactions can convert rhodanine derivatives into thiazolidines.
Substitution: Rhodanine derivatives can undergo nucleophilic substitution reactions, where the thioxo group is replaced by other nucleophiles.
Common Reagents and Conditions
Common reagents used in these reactions include oxidizing agents like hydrogen peroxide for oxidation, reducing agents like sodium borohydride for reduction, and nucleophiles like amines for substitution reactions . These reactions typically occur under mild conditions, such as room temperature and neutral pH.
Major Products Formed
The major products formed from these reactions include sulfoxides, sulfones, thiazolidines, and various substituted rhodanine derivatives .
Scientific Research Applications
Rhodanine, 3-veratryl-, has a wide range of scientific research applications:
Chemistry: It is used as a building block for the synthesis of various heterocyclic compounds.
Biology: Rhodanine derivatives exhibit significant antibacterial, antifungal, and antiviral activities.
Industry: Rhodanine derivatives are used in the development of chemosensors for detecting metal ions like Fe3+.
Mechanism of Action
The mechanism of action of Rhodanine, 3-veratryl-, involves its interaction with various molecular targets and pathways. Rhodanine derivatives are known to inhibit enzymes like carbonic anhydrase, which plays a crucial role in pH homeostasis . The inhibition of these enzymes disrupts cellular processes, leading to the compound’s pharmacological effects. Molecular docking studies have shown that rhodanine derivatives bind to the active sites of these enzymes, further elucidating their mechanism of action .
Comparison with Similar Compounds
Rhodanine, 3-veratryl-, can be compared with other rhodanine derivatives, such as:
Rhodanine-3-acetic acid: Used as a substrate in various syntheses and exhibits antibacterial activity.
Rhodanine-3-propionic acid: Similar to rhodanine-3-acetic acid but with a longer alkyl chain, affecting its geometry and interactions.
Rhodanine-3-butyric acid: Another homolog with a longer alkyl chain, used in the synthesis of various compounds.
Rhodanine, 3-veratryl-, is unique due to its specific substitution pattern, which can influence its biological activity and chemical reactivity. The presence of the veratryl group can enhance its pharmacological properties and make it a valuable compound for further research and development.
Properties
CAS No. |
23538-05-8 |
---|---|
Molecular Formula |
C12H13NO3S2 |
Molecular Weight |
283.4 g/mol |
IUPAC Name |
3-[(3,4-dimethoxyphenyl)methyl]-2-sulfanylidene-1,3-thiazolidin-4-one |
InChI |
InChI=1S/C12H13NO3S2/c1-15-9-4-3-8(5-10(9)16-2)6-13-11(14)7-18-12(13)17/h3-5H,6-7H2,1-2H3 |
InChI Key |
GEAXYSILQKHIOI-UHFFFAOYSA-N |
Canonical SMILES |
COC1=C(C=C(C=C1)CN2C(=O)CSC2=S)OC |
Origin of Product |
United States |
Disclaimer and Information on In-Vitro Research Products
Please be aware that all articles and product information presented on BenchChem are intended solely for informational purposes. The products available for purchase on BenchChem are specifically designed for in-vitro studies, which are conducted outside of living organisms. In-vitro studies, derived from the Latin term "in glass," involve experiments performed in controlled laboratory settings using cells or tissues. It is important to note that these products are not categorized as medicines or drugs, and they have not received approval from the FDA for the prevention, treatment, or cure of any medical condition, ailment, or disease. We must emphasize that any form of bodily introduction of these products into humans or animals is strictly prohibited by law. It is essential to adhere to these guidelines to ensure compliance with legal and ethical standards in research and experimentation.