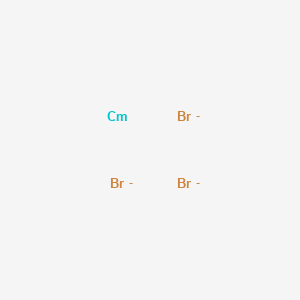
Curium bromide (244CmBr3)
- Click on QUICK INQUIRY to receive a quote from our team of experts.
- With the quality product at a COMPETITIVE price, you can focus more on your research.
Overview
Description
Curium tribromide is an inorganic compound with the chemical formula CmBr₃ It is a bromide salt of curium, a synthetic element in the actinide series Curium tribromide typically appears as a white or pale yellow-green solid and has an orthorhombic crystal structure
Preparation Methods
Curium tribromide can be synthesized through several methods:
-
Reaction of Curium Chloride and Ammonium Bromide
Reaction Conditions: This method involves reacting curium chloride (CmCl₃) with ammonium bromide (NH₄Br) in a hydrogen atmosphere at temperatures between 400°C and 450°C.
Reaction Equation: [ \text{CmCl}_3 + 3\text{NH}_4\text{Br} \rightarrow \text{CmBr}_3 + 3\text{NH}_4\text{Cl} ]
-
Reaction of Curium(III) Oxide and Hydrobromic Acid
Reaction Conditions: This method involves reacting curium(III) oxide (Cm₂O₃) with hydrobromic acid (HBr) at around 600°C.
Reaction Equation: [ \text{Cm}_2\text{O}_3 + 6\text{HBr} \rightarrow 2\text{CmBr}_3 + 3\text{H}_2\text{O} ]
These methods are typically used in laboratory settings due to the limited availability of curium and the specialized equipment required for handling radioactive materials .
Chemical Reactions Analysis
Curium tribromide undergoes various chemical reactions, including:
-
Oxidation and Reduction
- Curium tribromide can participate in redox reactions, where it can be reduced to lower oxidation states or oxidized to higher oxidation states.
-
Substitution Reactions
- In substitution reactions, the bromide ions in curium tribromide can be replaced by other halide ions or ligands. For example, reacting curium tribromide with a fluoride source can produce curium fluoride.
-
Hydrolysis
- Curium tribromide can hydrolyze in the presence of water, leading to the formation of curium hydroxide and hydrobromic acid.
Common reagents used in these reactions include halide salts, acids, and bases. The major products formed depend on the specific reaction conditions and reagents used .
Scientific Research Applications
Curium tribromide has several applications in scientific research:
-
Nuclear Science
- Curium tribromide is used in the study of nuclear reactions and the properties of actinide elements. Its radioactive nature makes it valuable for research in nuclear physics and chemistry.
-
Material Science
- The compound’s unique crystal structure and properties are of interest in material science, particularly in the development of new materials with specific electronic and magnetic properties.
-
Radiation Detection
- Curium tribromide can be used in radiation detection and measurement devices due to its radioactive properties. It is particularly useful in detecting alpha particles.
-
Medical Research
Mechanism of Action
The mechanism by which curium tribromide exerts its effects is primarily related to its radioactive nature. Curium emits alpha particles, which can interact with surrounding materials and biological tissues. The molecular targets and pathways involved in these interactions depend on the specific application and context. In nuclear reactions, curium tribromide can undergo fission, releasing a significant amount of energy .
Comparison with Similar Compounds
Curium tribromide can be compared with other similar compounds, such as:
-
Plutonium Tribromide (PuBr₃)
- Similar to curium tribromide, plutonium tribromide is an actinide bromide with comparable chemical properties. Both compounds have orthorhombic crystal structures and are used in nuclear research.
-
Americium Tribromide (AmBr₃)
- Americium tribromide shares similar properties with curium tribromide, including its use in radiation detection and nuclear science. americium has different nuclear properties and applications.
-
Cerium Tribromide (CeBr₃)
- Cerium tribromide is a lanthanide bromide with distinct properties compared to curium tribromide. It is used in scintillation detectors and has applications in medical imaging and environmental monitoring .
Curium tribromide’s uniqueness lies in its specific radioactive properties and its position in the actinide series, making it valuable for specialized scientific research and applications.
Properties
CAS No. |
14890-42-7 |
---|---|
Molecular Formula |
Br3Cm-3 |
Molecular Weight |
486.78 g/mol |
IUPAC Name |
curium;tribromide |
InChI |
InChI=1S/3BrH.Cm/h3*1H;/p-3 |
InChI Key |
HKCWOGXVUZTSCB-UHFFFAOYSA-K |
Canonical SMILES |
[Br-].[Br-].[Br-].[Cm] |
Origin of Product |
United States |
Disclaimer and Information on In-Vitro Research Products
Please be aware that all articles and product information presented on BenchChem are intended solely for informational purposes. The products available for purchase on BenchChem are specifically designed for in-vitro studies, which are conducted outside of living organisms. In-vitro studies, derived from the Latin term "in glass," involve experiments performed in controlled laboratory settings using cells or tissues. It is important to note that these products are not categorized as medicines or drugs, and they have not received approval from the FDA for the prevention, treatment, or cure of any medical condition, ailment, or disease. We must emphasize that any form of bodily introduction of these products into humans or animals is strictly prohibited by law. It is essential to adhere to these guidelines to ensure compliance with legal and ethical standards in research and experimentation.